Evaluation of miR-122-regulated suicide gene therapy for hepatocellular carcinoma in an orthotopic mouse model
Introduction
Hepatocellular carcinoma (HCC) is one of the most common cancers worldwide and the third most common cause of death from cancers (1). Unresectable HCC lacks effective therapy and new therapeutic modalities are urgently needed (2,3). Suicide gene therapy is a rational, potential therapeutic approach for the treatment of HCC and liver metastases of digestive tumors (2,3). One specific suicide gene therapy strategy is the virus-mediated transduction of herpes simplex virus (HSV) thymidine kinase (TK) gene followed by the systemic administration of ganciclovir (GCV). The TK gene product phosphorylates GCV to activate its potential to terminate DNA synthesis, leading to cell death (4,5). Not only were cells transduced by TK gene killed, but also TK-negative cells were killed as a result of a “bystander effect” (6,7). Among the commonly used gene transfer vectors, adenovirus (Ad) vectors are particularly attractive for in vivo gene therapy of cancers (8). Several studies have demonstrated the efficacy of intratumoral injection of Ad-mediated TK/GCV system for the treatment of HCC (9-13). In the model of HCC, tumors can be regressed and even eliminated in vivo by the TK/GCV system in an Ad vector dosage-dependent manner. One of the main obstacles limiting the application of this therapy is toxic side effects affecting normal liver tissue. Because of the high hepatic tropism of Ad vectors, an unwanted spread of vectors and TK expression in peritumoral and remote liver tissue lead to severe hepatic damage (12-15). The severe hepatoxicity occurred in TK/GCV-treated animals especially at effective dosage and resulted in the failure of therapy. Stringent regulation of transgene expression is required to increase the safety and efficacy of suicide gene therapy for HCC.
A vast post-transcriptional regulatory network mediated by microRNA (miRNA), a class of abundant small noncoding RNAs, provides improved control over gene expression (16). Mature miRNAs perfectly, or near perfectly, bind to complementary sequences in the 3'-untranslated regions (UTRs) of target mRNAs and cause either translational repression or mRNA degradation (17). Tissue-specific miRNA expression has been well clarified (18) and provides an opportunity to regulate transgene expression from therapeutic nucleic acids and viruses. miRNA target sequences have been inserted into the 3'-UTR of transgene to restrict its expression in a specific cell or tissue (16). MicroRNA-122 (miR-122) is the most abundant liver-specific miRNA (18,19). Studies show that miR-122 is significantly down-regulated in 50-70% of human primary HCC and in all HCC-derived cell lines (20-27). Since the decrease in hepatic miR-122 was a tumor-specific event, we hypothesized inserting miR-122 target sequences (miR-122T) into the 3'-UTR of the TK transcription cassette would improve tumor-specific expression by repressing TK expression in non-malignant liver tissue, and as a result, reduce hepatotoxicity, while keeping the antitumor effects. In this study, we have constructed Ad vectors expressing miR-122-regulated TK (Ad-TK-122T) and report genes. By intratumoral delivery of these Ad vectors to a murine orthotopic HCC model based on the miR-122-deficient murine HCC cell line Hepa 1-6 (26), we have researched miR-122-regulated gene expression in HCC and liver, the anti-tumor effects and hepatotoxicity of miR-122-reglated suicide gene therapy for HCC.
Materials and methods
Cell lines
HEK-293, HeLa, Huh7 and Hepa 1-6 cells were purchased from the American Type Culture Collection. All cells were grown in DMEM with 10% fetal bovine serum, 1% penicillin/streptomycin, and 1% L-glutamine, cultured in a humidified incubator at 37 °C with 5% CO2.
Animals
Five-week-old male C57BL/6 mice were purchased from HFK bioscience Co., Ltd. (Beijing, China) and housed in the Animal Care Center of the Chinese Center for Disease Control and Prevention under pathogen-free conditions. All procedures involving animals and their care were conducted in conformity with institutional guidelines, which are in compliance with national and international laws and guidelines.
Plasmids and Ad vectors
The AdMax™ system was used to produce all Ad vectors for this research. Enhanced green fluorescent protein (EGFP), firefly luciferase (Fluc) and TK gene were cloned into the EcoR I and Bgl II sites of pDC316 which was a shuttle plasmid containing an expression cassette utilizing the CMV promoter. In this way, the EGFP, Fluc and TK expressing plasmids, named pDC316-EGFP, pDC316-Fluc and pDC316-TK, were constructed. Oligonucleotides containing four copies of miR-122 completely binding sites (forward: 5'-AGCTTCAAACACCATTGTCACACTCCAAGACCAAACACCATTGTCACACTCCAAGACCAAACACCATTGTCACACTCCAAGACCAAACACCATGTCACACTCCAGAGCT-3', reverse: 5'-CTGGAGTGTGACAATGGTGTTTGGTCTTGGAGTGTGACAATGGTGTTTGGTCTTGGAGTGTGACAATGGTGTTTGGTCTTGGAGTGTGACAATGGTGTTTGA-3') (the miR-122T sequences were underlined) were annealed at 95 °C, cooled and ligated into pDC316-EGFP, pDC316-Fluc or pDC316-TK cleaved with Hind III and Sac I. In this way, miR-122-regulated EGFP, Fluc, or TK expression plasmids, named pDC316-EGFP-122T, pDC316-Fluc-122T and pDC316-TK-122T, were constructed. All plasmids were cotransfected with an Ad genomic plasmid into HEK293 cells using the AdMax™ system to produce Ad-EGFP, Ad-EGFP-122T, Ad-Fluc, Ad-Fluc-122T, Ad-TK and Ad-TK-122T (Figure 1), and further propagated in HEK293 cells. All Ad vectors were purified by two rounds of cesium chloride density gradient ultracentrifugation. Viral particle (vp) titers were determined by spectrophotometry at OD260.
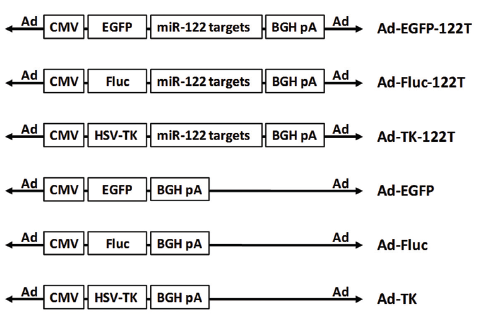
In vitro gene expression analysis
HeLa, Huh7 or Hepa 1-6 cells were infected with Ad-EGFP-122T or Ad-EGFP respectively. Forty-eight hours later, EGFP expression was observed in fluorescence microscope. Aforementioned cells were infected with Ad-Fluc-122T or Ad-Fluc respectively. Forty-eight hours later, relative light units (RLU) in cultured cells were measured using the commercial luciferase assay system (Promega), according to the manufacturer’s instructions in a luminometer.
In vitro MTT assay
HeLa, Huh7 or Hepa 1-6 cells were infected with Ad-TK-122T or Ad-TK respectively and cultured in 96-well plates with 150 µL complete medium in the presence of different concentrations of GCV for 5 d. On day 5, 20 µL 3-(4,5)-dimethylthiahiazo(-z-y1)-3,5-di-phenytetrazoliumromide (MTT) solution (5 mg/mL) was added to each well, and incubation at 37 °C in 5% CO2 was continued for 3 h. Supernatants were removed and 100 µL dimethylsulfoxide (DMSO) was added to each well. After 30 min incubation, absorbances were measured using a microplate spectraphotometer equipped with a 570 nm filter.
Establishment an orthotopic transplant mouse model of HCC
Five-week-old male C57BL/6 mice were anesthetized with pentobarbital sodium (75 mg/kg). A transverse incision was made across the xyphoid process and extended approximately 2 cm. Hepa 1-6 cells (1×106/mouse) in 10 µL were injected into the front of the left upper liver lobe, using a 30-gauge needle. The lobe was returned to the abdomen and the incision was closed with sutures.
Intratumoral injection of Ad vectors
Ten days after injection of Hepa 1-6 cells in C57BL/6 mice, when the tumor volume was 50-100 mm3, surgery was performed as described earlier and 10 µL of Ad vector was injected slowly and carefully into the centre of the tumor, using a 30-gauge needle.
In vivo gene expression analysis
Two days after Ad-EGFP or Ad-EGFP-122T, Ad-Fluc or Ad-Fluc-122T injected into tumors in HCC models, as described earlier, tumor and liver tissues were isolated and frozen sections were made. The EGFP expression was observation in fluorescence microscope and hematoxylin & eosin (HE) stain was also made to differentiate liver and tumor tissues. The whole liver tissue or tumor tissue was grinded respectively and RLU was estimated using the commercial luciferase assay system, according to the manufacturer’s instructions in a luminometer.
TK/GCV treatment and in vivo antitumor effect study
Twelve hours after Ad-TK or Ad-TK-122T injected into tumors in HCC models, as described earlier, the mice were received intraperitoneal injections of GCV (35 mg/kg) twice every day for 5 d. Ten days after TK/GCV treatment, the mice were scarified and tumor growth was monitored by calculating the tumor volume from caliper measurements. Tumor volumes were calculated according to the formula: (tumor volume; mm3) = (major axis; mm) × (minor axis; mm)2/2.
Measurement of serum alanine aminotransferase (ALT)
Ten days after TK/GCV was performed, blood samples were collected through tail vein bleeding and allowed to clot (15 min, room temperature) and spun at 1,200 g for 10 min to collect the serum. The serum ALT levels were determined using an ALT kit (Human GmbH) according to the manufacturer’s instructions in an automatic chemistry analyzer.
Histology
The liver lobes from each mouse were immersed in 10% buffered formalin overnight at room temperature, embedded in wax and sectioned using a vibratome. Sections were stained with HE and analyzed by light microscopy at 200× magnification.
Statistical analysis
Data were expressed as . Significance was evaluated using t-test and denoted on the graphs as *P<0.05, **P<0.01.
Results
In vitro performance of miR-122-regulated report genes and TK/GCV system
To assess in vitro miR-122-regulated transgene expression mediated by Ad vectors, Ad-EGFP-122T or Ad-EGFP was infected into HeLa, Huh7 or Hepa 1-6 cells at doses of 5,000, 1,000 or 1,000 vp/cell respectively. EGFP expression was analyzed by fluorescence microscopy 48 h later. As shown in Figure 2A, in Huh7 cells which are known to be miR-122-positive (19,26), the expression of EGFP was down-regulated dramatically. In contrast, the presence of miR-122T did not affect the EGFP expression in miR-122-negative HeLa cells (26,28). Moreover, insertion of miR-122T did not reduce the EGFP expression in Hepa 1-6 cells, suggesting the murine HCC cell line Hepa 1-6 was lack of miR-122 expression. This result was in conformity with previous studies which reported miR-122 expressed at very low levels in Hepa 1-6 cells (26). We also evaluated the miR-122-regulated Fluc expression in Huh7, HeLa and Hepa 1-6 cells by using Ad-Fluc-122T and Ad-Fluc. Cells were harvested 48 h after Ad infection and luciferase activities in the cells were detected (Figure 2B). As compared to Ad-Fluc, 8.06-fold repression of luciferase activity was found in Huh7 cells infected with Ad-Fluc-122T (P<0.05). However, there was no inhibition of luciferase activities in HeLa and Hepa 1-6 cells infected with Ad-Fluc-122T. These results suggested miR-122’s negative regulation was effective and specific in vitro for genes containing miR-122T.
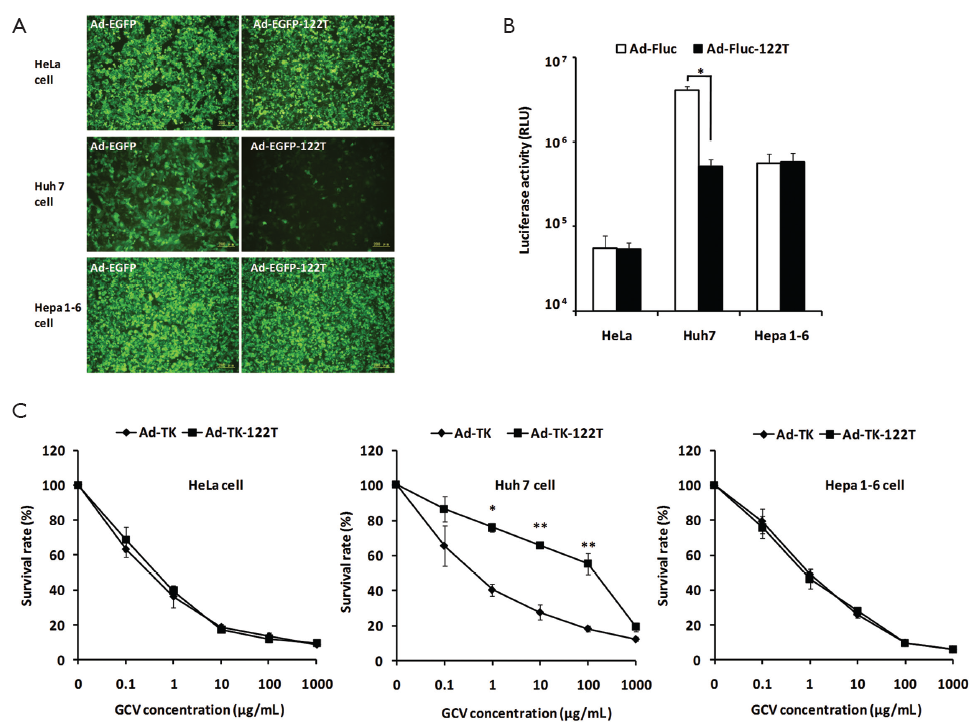
To evaluate if miR-122 regulation would protect miR-122-positive cells from conditional killing, we infected respectively Ad-TK-122T or Ad-TK into Huh7, HeLa or Hepa 1-6 cells in the presence of GCV, and tested the viable cell number by MTT assay. As shown in Figure 2C, Ad-TK-122T-infected Huh7 cells were much less injured in the presence of GCV at doses of 1-100 µg/mL, compared to Ad-TK-transfected cells (P<0.05, P<0.01). However, HeLa cells infected with Ad-TK-122T or Ad-TK were both effectively killed by TK/GCV system. For Hepa 1-6 cells, infection with Ad-TK-122T resulted in the same cell death as infection with Ad-TK. These data indicated that inhibiting expression of TK gene by miR-122 down-regulation was a feasible way to protect cells endogenously expressing miR-122 from killing by GCV.
In vivo examining miR-122-regulated transgene expression
Next, we evaluated the potency of miR-122 regulation in vivo. Ad-EGFP-122T at the dose of 1×1010 vp or the control Ad-EGFP at the same amount was injected intratumorally in the orthotopic Hepa 1-6 tumor model. After 2 d, we found that mice treated with Ad-EGFP showed a high level of EGFP expression not only in tumor but also in peritumoral liver tissue (Figure 3A). In contrast, mice treated with Ad-EGFP-122T showed no EGFP expression in peritumoral liver tissue, compare to the high level of EGFP expression in tumor tissue (Figure 3B). We also evaluated the repression capabilities of miR-122 by using Fluc gene. Two days after intratumoral injection of 1×1010 vp of Ad-Fluc, luciferase activities had been detected not only in tumor tissue but also in peritumoral and remote liver tissue (Figure 3C). The average luciferase activity in liver tissue (586,889±325,833 RLU) was higher than that in tumor tissue (323,456±23,778 RLU). The luciferase activity ratio of tumor to liver tissue was 0.55:1.00 (Figure 3D). In contrast to Ad-Fluc, intratumoral injection of Ad-Fluc-122T resulted in significant reduction of Fluc expression in liver tissue (50,354±22,802 RLU) (P<0.05), but mediated nearly the same level of Fluc expression in tumor tissue (307,307±77,497 RLU) (Figure 3C) as that of Ad-Fluc. The luciferase activity ratio of tumor to liver tissue was 6.10:1.00 (Figure 3D). These results suggested that the expression of transgene containing miR-122T was inhibited strongly in the liver, but the inserting of miR-122T did not influence the transgene expression in the Hepa 1-6 tumor. As a result, the insertion of miR-122T acquires an 11.09-fold improvement of tumor-specific transgene expression.
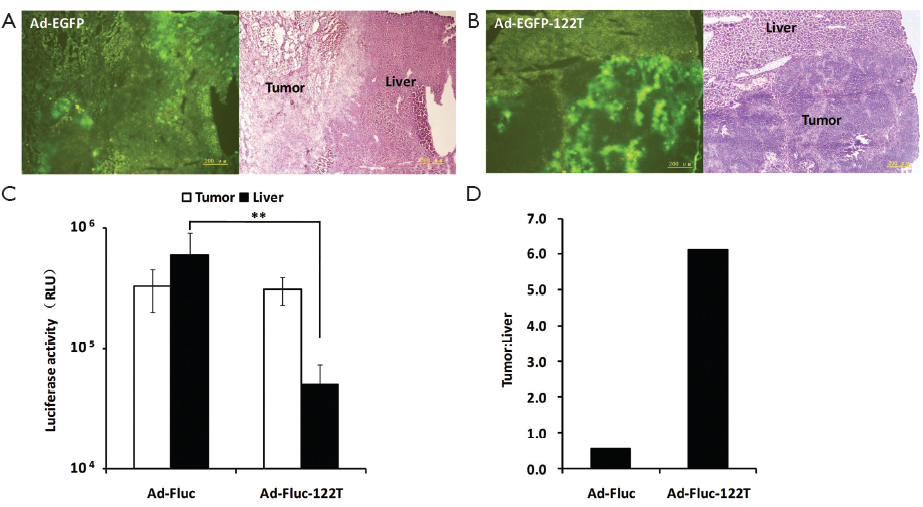
Antitumor effects of miR-122 regulated TK/GCV system
Next, we evaluated the antitumor effects of adenovirus-mediated miR-122 regulated TK/GCV system. Ad-TK-122T, Ad-TK or Ad-Fluc at doses of 1×109, 3×109 or 1×1010 vp and normal saline (NS) control was intratumorally injected into orthotopic Hepa 1-6 tumor models following GCV treatment for 5 d. Ten days after TK/GCV treatment, the mice were scarified and tumor growth was monitored (Figure 4A). The results showed that injection of Ad-TK-122T and Ad-TK at the doses of 1×109 and 3×109 vp did not lead to significant antitumor effects as compared to Ad-Fluc injected control (Figure 4B). When the dose of Ad vectors increased to 1×1010 vp, the tumors of mice injected with Ad-TK and Ad-TK-122T were significantly repressed, compared to the Ad-Fluc injected control (P<0.01) (Figure 4B). There were no significant differences in the antitumor activity between Ad-TK-122T and Ad-TK. These results suggested that intratumoral injection of Ad-TK-122T or Ad-TK resulted in a vector dosage-dependent regression in HCC. The insertion of miR-122T does not influence the antitumor effects of suicide gene therapy for the Hepa 1-6 tumor model by intratumoral injection of TK-expressing Ad vectors.
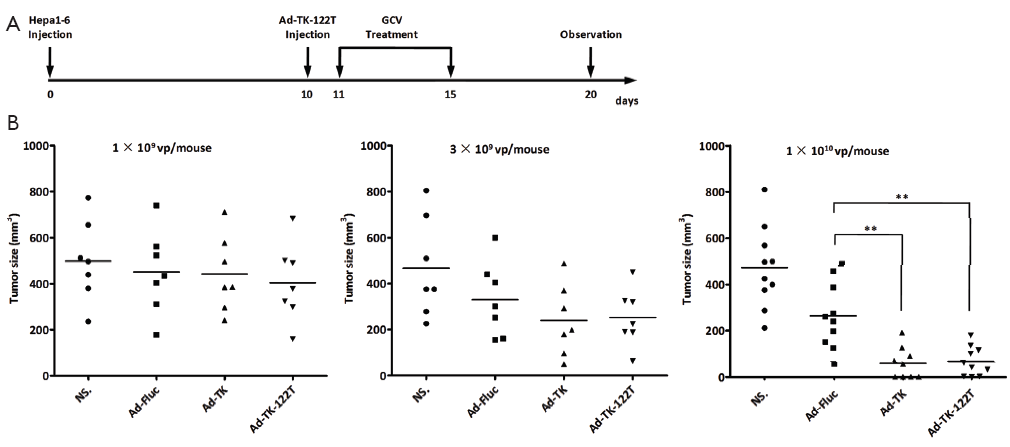
Assessment of hepatotoxicity of miR-122 regulation TK/GCV system
To examine the hepatotoxicity of the adenovirus-mediated miR-122-regulated TK/GCV system, examinations of ALT, relative body weight and histology were performed 10 d after TK/GCV treatment. Mice administered Ad-TK at doses of 3×109 and 1×1010 vp showed significantly increased ALT levels (1.2 and 4.0 times higher than control mice treated with Ad-Fluc at the same doses, P<0.05 and P<0.01, Figure 5A). Mice administered Ad-TK-122T at doses of 3×109 and 1×1010 vp showed no significant increase of serum ALT as compared to the control mice treated with Ad-Fluc. Moreover, whereas mice that treated with Ad-TK at 1×1010 vp showed a significant reduction in body weight compared to the Ad-Fluc control (P<0.01), mice treated with Ad-TK-122T at the same dose did not show any significant loss in body weight (Figure 5B). Histological analysis showed a dramatic difference between mice administered Ad-TK and those administered Ad-TK-122T at the dose of 1×1010 vp (Figure 5C). Injection of Ad-TK resulted in vacuolation, hemorrhaging and abnormal nuclear morphology in mouse livers. However, livers from mice administered Ad-TK-122T showed very little pathological changes. In addition, while one mouse in the study which received 1×1010 vp of Ad-TK became hunched and immobile, and died after 9 d, all mice treated with Ad-TK-122T or Ad-Fluc were alive during the experiment. These results indicated that insertion of miR-122T effectively avoided the hepatotoxicity caused by intratumoral delivery of adenovirus-mediated TK/GCV suicide gene therapy.
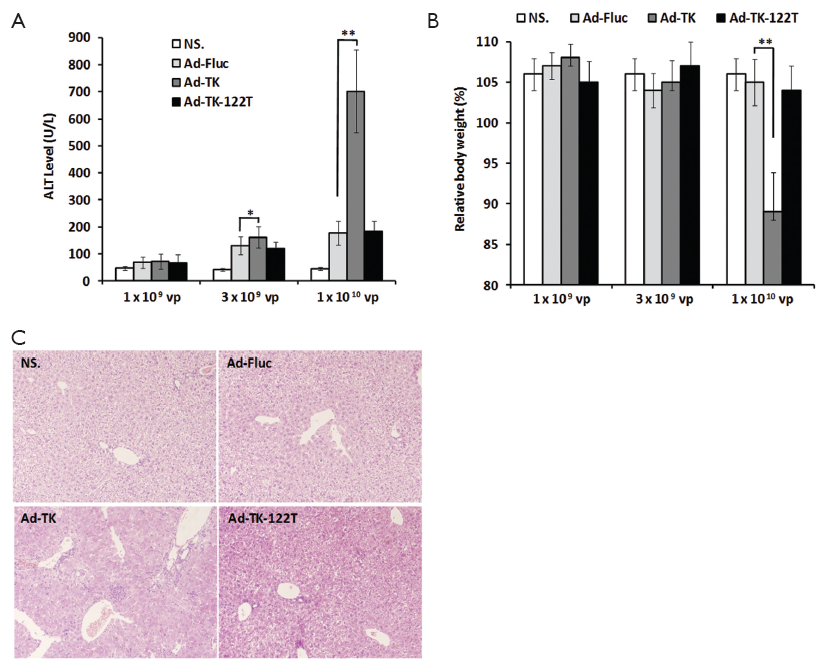
Discussion
miR-122 is highly and selectively expressed in hepatocytes and attracts much attention to be considered as elements for hepatic specific targeting viral vector (18,19,29,30). Several studies have confirmed that insertion of miR-122T is an effective strategy to specifically down-regulate gene expression in liver. By incorporating miR-122T in the 3'-UTR of the E1A gene, the recombinant Ad replicated normally in other cells but not in cells of hepatic origin (31,32). miR-122T could selectively down-regulate adenoviral luciferase expression in the liver and improve tumor-specific transgene expression in a human fibrosarcoma model (33). miR-122T incorporated in adeno-associated virus (AAV) vectors efficiently inhibited transgene expression in the liver (34). Adenovirus-mediated suicide gene therapy using the TK gene with miR-122T was reported to prevent hepatotoxicity when the Ad vector was injected locally into melanoma B16 tumor-bearing mice (35). miR-122 was significantly down-regulated in 50% of HCC compared to non-malignant liver tissue from the same individuals and was not detectable in HCC cell lines such as human (HepG2, Hep3B) or mouse (Hepa 1-6) cells and expressed at a significantly low level in human (Huh7) and rat (H4) cells compared to the hepatocytes (20-24). The different expression profiles in HCC as well as the specificity and abundance in liver make miR-122 attractive to be considered as elements for tumor-specific gene expression. In this study, we explored the efficiency and safety of suicide gene therapy for HCC in an orthotopic Hepa 1-6 tumor model based on intratumoral injection Ad vectors that mediated miR-122-regulated TK expression.
By inserting four copies of miR-122T sequences in the 3'-UTR of EGFP, Fluc and TK genes, the recombinant Ad expressing miR-122-regulated report gene or suicide gene have been constructed. We confirmed that miR-122’s negative regulation was effective and specific in vitro for genes containing miR-122T in the 3'-UTR; miR-122 down-regulation could protect cells endogenously expressing miR-122 (Huh7) from killing and did not affect killing the miR-122-negitive cells (Hepa 1-6 and HeLa). We established the mouse HCC models by orthotopic injection of miR-122-deficient Hepa 1-6 cells and researched the performance of intratumoral injection of Ad vectors expressing miR-122-regulated report genes. We found intratumoral injection of Ad-EGFP or Ad-Fluc of less 1/5 of tumor volume would result in report gene expressing not only in tumor but also in peritumoral and remote liver tissue. The average report gene expression levels in liver tissue were even higher than those in tumor tissue. In contrast, when Ad-EGFP-122T or Ad-Fluc-122T was injected intratumorally, the report gene expression was not altered in tumor tissue but was repressed significantly in liver tissue. miR-122-regulation resulted in an 11.09-fold improvement of Hepa 1-6 tumor-specific transgene expression. It suggested that insertion of miR-122T is an effective strategy to realize the tumor-specific tansgene expression for intratumoral injection Ad vectors in the orthotopic miR-122-down-regulated HCC model. The further results showed that the tumor growth of mice after injection of Ad-TK-122T and Ad-TK were both inhibited in a vector dose-dependent manner. Treatment with 1×1010 vp of Ad-TK or Ad-TK-122T acquired significant antitumor effects. The insertion of miR-122T did not affect the antitumor effects in the Hepa 1-6 tumor model. While intratumoral injection of Ad-TK at the effective dose (1×1010 vp) resulted in fatal hepatotoxicity, no significant difference in hepatotoxicity was found at mice administrated with Ad-TK-122T at this dose as compared with those treated with a control Ad vector. These results indicated that suicide gene therapy based on intratumoral injection of Ad vectors encoding miR-122-regulated TK gene was safer and more efficient by reducing hepatotoxicity perfectly in the HCC model.
Several factors contribute to the effective antitumor response and safety of adenovirus-mediated miR-122-regulated TK gene therapy in this study. Hepa 1-6 cells which highly express coxsakievirus and adenovirus receptor (CAR) can be effectively infected by Ad vectors (36). Due to the lack of miR-122 in Hepa 1-6 cells, TK gene expression has not be impaired by insertion of miR-122T. These features ensure the sufficient TK gene expression in Hepa 1-6 tumor. Although liver tropism of Ad vectors could induce high transduction of TK gene in hepatocytes, the abundance of miR-122 in the live induced strong miR-122’s negative regulation which led to dramatic repression of TK expression, avoiding hepatotoxicity.
A phase I clinical trial of TK-based suicide gene therapy in HCC indicated that Ad-TK can be safely administered by intratumoral injection to patients with HCC up to 2×1012 vp per patient (37). And an ealier phase I clinical trial indicated that Ad-TK can be safely administered by intratumoral injection in patients with hepatic metastases at doses up to 1×1013 vp per patient (38). The doses of Ad vectors used in our study were higher than those in clinical trials. These indicate that the miR-122-regulated TK/GCV system could be used to increase the injected dose of TK-expressing Ad vectors and thereby result in greater antitumor effects in clinical trials for HCC.
It should be pointed out that the miR-122-regulated TK expression system is not fit for cases of HCC with high-level expression of miR-122 which would result in insufficient TK expression levels in tumor and then treatment failure. Although miR-122’s down-regulation is a common feature in human and mouse HCC, different cases may vary greatly in miR-122’s levels. However, due to the liver-specific expression of miR-122, the miR-122-regulated TK expression system could be used to eliminate hepatic metastatic tumors in which miR-122 is negative. Beside suicide gene therapy, miR-122 regulation could be also used to avoid liver toxicity when cytotoxic and pro-apoptotic genes such as FASL, TNF-α and TRAIL are used as therapeutic genes in gene therapy for HCC.
In conclusion, miR-122-regulated TK expression achieves effective antitumor effects and increases the safety of adenovirus-mediated TK/GCV gene therapy for miR-122-down-regulated HCC. Insertion of miR-122T makes it possible to increase the dose of adenovirus-mediated TK/GCV gene therapy for HCC to get more effective therapeutic effect. Intratumoral injection of adenovirus-mediated miR-122-regulated TK may be useful in the treatment of miR-122-down-regulated HCC.
Acknowledgements
This work was funded by the National 863 Program (No. 2012AA020810) and Beijing city strategic emerging industry (No. Z121102002912040).
Disclosure: The authors declare no conflict of interest.
References
- Parkin DM, Bray F, Ferlay J, et al. Global cancer statistics, 2002. CA Cancer J Clin 2005;55:74-108. [PubMed]
- Schmitz V, Qian C, Ruiz J, et al. Gene therapy for liver diseases: recent strategies for treatment of viral hepatitis and liver malignancies. Gut 2002;50:130-5. [PubMed]
- Kidner T, Dai M, Adusumilli PS, et al. Advances in experimental and translational research in the treatment of hepatocellular carcinoma. Surg Oncol Clin N Am 2008;17:377-89. [PubMed]
- Moolten FL. Tumor chemosensitivity conferred by inserted herpes thymidine kinase genes: paradigm for a prospective cancer control strategy. Cancer Res 1986;46:5276-81. [PubMed]
- Moolten FL. Drug sensitivity (“suicide”) genes for selective cancer chemotherapy. Cancer Gene Ther 1994;1:279-87. [PubMed]
- Freeman SM, Abboud CN, Whartenby KA, et al. The “bystander effect”: tumor regression when a fraction of the tumor mass is genetically modified. Cancer Res 1993;53:5274-83. [PubMed]
- Mesnil M, Yamasaki H. Bystander effect in herpes simplex virus-thymidine kinase/ganciclovir cancer gene therapy: role of gap-junctional intercellular communication. Cancer Res 2000;60:3989-99. [PubMed]
- Kovesdi I, Brough DE, Bruder JT, et al. Adenoviral vectors for gene transfer. Curr Opin Biotechnol 1997;8:583-9. [PubMed]
- Qian C, Idoate M, Bilbao R, et al. Gene transfer and therapy with adenoviral vector in rats with diethylnitrosamine-induced hepatocellular carcinoma. Hum Gene Ther 1997;8:349-58. [PubMed]
- Sa Cunha A, Bonte E, Dubois S, et al. Inhibition of rat hepatocellular carcinoma tumor growth after multiple infusions of recombinant Ad.AFPtk followed by ganciclovir treatment. J Hepatol 2002;37:222-30. [PubMed]
- Mizuguchi H, Hayakawa T. Enhanced antitumor effect and reduced vector dissemination with fiber-modified adenovirus vectors expressing herpes simplex virus thymidine kinase. Cancer Gene Ther 2002;9:236-42. [PubMed]
- Gerolami R, Cardoso J, Lewin M, et al. Evaluation of HSV-tk gene therapy in a rat model of chemically induced hepatocellular carcinoma by intratumoral and intrahepatic artery routes. Cancer Res 2000;60:993-1001. [PubMed]
- Bilbao R, Gérolami R, Bralet MP, et al. Transduction efficacy, antitumoral effect, and toxicity of adenovirus-mediated herpes simplex virus thymidine kinase/ganciclovir therapy of hepatocellular carcinoma: the woodchuck animal model. Cancer Gene Ther 2000;7:657-62. [PubMed]
- Tjuvajev JG, Chen SH, Joshi A, et al. Imaging adenoviral-mediated herpes virus thymidine kinase gene transfer and expression in vivo. Cancer Res 1999;59:5186-93. [PubMed]
- Brand K, Arnold W, Bartels T, et al. Liver-associated toxicity of the HSV-tk/GCV approach and adenoviral vectors. Cancer Gene Ther 1997;4:9-16. [PubMed]
- Brown BD, Gentner B, Cantore A, et al. Endogenous microRNA can be broadly exploited to regulate transgene expression according to tissue, lineage and differentiation state. Nat Biotechnol 2007;25:1457-67. [PubMed]
- Marquez RT, McCaffrey AP. Advances in microRNAs: implications for gene therapists. Hum Gene Ther 2008;19:27-38. [PubMed]
- Lagos-Quintana M, Rauhut R, Yalcin A, et al. Identification of tissue-specific microRNAs from mouse. Curr Biol 2002;12:735-9. [PubMed]
- Chang J, Nicolas E, Marks D, et al. miR-122, a mammalian liver-specific microRNA, is processed from hcr mRNA and may downregulate the high affinity cationic amino acid transporter CAT-1. RNA Biol 2004;1:106-13. [PubMed]
- Kutay H, Bai S, Datta J, et al. Downregulation of miR-122 in the rodent and human hepatocellular carcinomas. J Cell Biochem 2006;99:671-8. [PubMed]
- Gramantieri L, Ferracin M, Fornari F, et al. Cyclin G1 is a target of miR-122a, a microRNA frequently down-regulated in human hepatocellular carcinoma. Cancer Res 2007;67:6092-9. [PubMed]
- Fornari F, Gramantieri L, Giovannini C, et al. MiR-122/cyclin G1 interaction modulates p53 activity and affects doxorubicin sensitivity of human hepatocarcinoma cells. Cancer Res 2009;69:5761-7. [PubMed]
- Bai S, Nasser MW, Wang B, et al. MicroRNA-122 inhibits tumorigenic properties of hepatocellular carcinoma cells and sensitizes these cells to sorafenib. J Biol Chem 2009;284:32015-27. [PubMed]
- Burchard J, Zhang C, Liu AM, et al. microRNA-122 as a regulator of mitochondrial metabolic gene network in hepatocellular carcinoma. Mol Syst Biol 2010;6:402. [PubMed]
- Li C, Wang Y, Wang S, et al. Hepatitis B virus mRNA-mediated miR-122 inhibition upregulates PTTG1-binding protein, which promotes hepatocellular carcinoma tumor growth and cell invasion. J Virol 2013;87:2193-205. [PubMed]
- Jopling CL, Yi M, Lancaster AM, et al. Modulation of hepatitis C virus RNA abundance by a liver-specific MicroRNA. Science 2005;309:1577-81. [PubMed]
- Budhu A, Jia HL, Forgues M, et al. Identification of metastasis-related microRNAs in hepatocellular carcinoma. Hepatology 2008;47:897-907. [PubMed]
- Henke JI, Goergen D, Zheng J, et al. microRNA-122 stimulates translation of hepatitis C virus RNA. EMBO J 2008;27:3300-10. [PubMed]
- Zhang G, Wang Q, Xu R. Therapeutics Based on microRNA: A New Approach for Liver Cancer. Curr Genomics 2010;11:311-25. [PubMed]
- Filipowicz W, Grosshans H. The liver-specific microRNA miR-122: biology and therapeutic potential. Prog Drug Res 2011;67:221-38. [PubMed]
- Ylösmäki E, Hakkarainen T, Hemminki A, et al. Generation of a conditionally replicating adenovirus based on targeted destruction of E1A mRNA by a cell type-specific MicroRNA. J Virol 2008;82:11009-15. [PubMed]
- Cawood R, Chen HH, Carroll F, et al. Use of tissue-specific microRNA to control pathology of wild-type adenovirus without attenuation of its ability to kill cancer cells. PLoS Pathog 2009;5:e1000440. [PubMed]
- Card PB, Hogg RT, Gil Del Alcazar CR, et al. MicroRNA silencing improves the tumor specificity of adenoviral transgene expression. Cancer Gene Ther 2012;19:451-9. [PubMed]
- Qiao C, Yuan Z, Li J, et al. Liver-specific microRNA-122 target sequences incorporated in AAV vectors efficiently inhibits transgene expression in the liver. Gene Ther 2011;18:403-10. [PubMed]
- Suzuki T, Sakurai F, Nakamura S, et al. miR-122a-regulated expression of a suicide gene prevents hepatotoxicity without altering antitumor effects in suicide gene therapy. Mol Ther 2008;16:1719-26. [PubMed]
- Han SY, Lee YJ, Jung HI, et al. Gene transfer using liposome-complexed adenovirus seems to overcome limitations due to coxsackievirus and adenovirus receptor-deficiency of cancer cells, both in vitro and in vivo. Exp Mol Med 2008;40:427-34. [PubMed]
- Sangro B, Mazzolini G, Ruiz M, et al. A phase I clinical trial of thymidine kinase-based gene therapy in advanced hepatocellular carcinoma. Cancer Gene Ther 2010;17:837-43. [PubMed]
- Sung MW, Yeh HC, Thung SN, et al. Intratumoral adenovirus-mediated suicide gene transfer for hepatic metastases from colorectal adenocarcinoma: results of a phase I clinical trial. Mol Ther 2001;4:182-91. [PubMed]