Preclinical characterization and validation of a dual-labeled trastuzumab-based imaging agent for diagnosing breast cancer
Introduction
Microdose administration of therapeutic monoclonal antibodies that are labeled for diagnostic imaging at a subtherapeutic dose may provide a method for evaluating the spatial and temporal tissue heterogeneity of clinically relevant disease markers for proper selection of molecular therapies and their associated regimens (1). Recently, we have demonstrated the feasibility of multimodality imaging in noninvasively detecting malignant breast tumors with both nuclear and near-infrared (NIR) optical imaging (2-4). Results suggested that the high-energy photons emitted from the radionuclide enable deep interrogation of tissues, whereas high fluorescent photon count rates of NIR fluorophores, and their ability to be repeatedly activated, enable non-invasive, real-time imaging as well as potential image-guided surgery followed by rapid and accurate surgical pathology.
Trastuzumab (Herceptin™, Genentech, South San Francisco, USA) is a humanized antibody specifically targeting human epidermal growth factor receptor 2 (HER2), which is overexpressed in 25-30% of breast cancers, and in adenocarcimomas of the ovary, prostate, lung and gastrointestinal tract (5). Overexpression of HER2, associated with a poor prognosis and aggressive tumor attributes, makes this target suitable for molecular imaging and therapy (6,7). To date, the majority of molecular imaging of HER2 is based upon trastuzumab targeting using nuclear imaging techniques, and no NIR optical imaging agent has been used clinically for this application (8-11).
In order to validate a dual-labeled imaging agent for breast cancer diagnostic use, we dual-labeled trastuzumab with a NIR fluorophore, IRDye 800CW, and diethylenetriamine pentaacetic acid (DTPA) for chelation of Indium-111. The potential for employing both fluorescence and nuclear agents in breast cancer has been demonstrated in preclinical animal models (3). However, even though the fluorescence signal intensity observed in the tumor region of the nude mice was significantly higher than that in the rest of the body, the tumor-to-muscle ratio, calculated from both radioactive and fluorescence data, was as low as 2.25 (9). This fact can definitely hamper the progress of [111In]-DTPA-trastuzumab-IRDye 800 in moving “from bench to bedside”. Moreover, issues, such as purity evaluation of a dual-labeled conjugate and validation of immunoreactivity assays, must be resolved for successful translational studies.
In this study, we put in our effort to optimize dual-labeled conjugate preparation to improve bioreactivity and biodistribution. In addition, we validated methods that can assist in the translation of optical imaging agents into “first-in-humans” clinical study.
Materials and methods
Material and instrumentation
Trastuzumab (Herceptin™, Genentech South, USA) was purchased from clinical pharmacy for research purposes. The NIR fluorescent IRDye 800CW was from Li-CorBioscence (Lincoln, USA), and p-SCN-Bn-DTPA from Macrocyclics (Dallas, USA). [111In]Cl3 was obtained from Perkin-Elmer Life Sciences (Waltham, USA). Ultrafiltration membranes with a 50 kD molecular weight cutoff were from Millipore (Danvers, USA). Tris-glycine polyacrylamide gels were from Bio-Rad (Hercules, USA). Thin layer chromatography (TLC) plates were obtained from EMD Chemicals (Gibbstown, USA). SKBR-3 cells were obtained from the American Type Culture Collection (Manassas, USA); GIBCO culture reagents were purchased through Invitrogen (Carlsbad, USA). All other chemicals were obtained from Sigma-Aldrich (St. Louis, USA).
Preparation of radionuclide and optical-labeled trastuzumab conjugates
[111In]-DTPA-trastuzumab-IRDye 800 was synthesized by a three-step procedure, where trastuzumab was conjugated to IRDye 800CW and then to DTPA and finally radiolabeled with In-111 (Figure 1).
Determination of average number of chelates and dyeper antibody
The molar ratio of DTPA-to-protein, “n”, was determined using the methods described by Hnatowich et al. (12) DTPA-trastuzumab-IRDye 800 was diluted in a mixture of phosphate buffered saline (PBS):methanol (1:1), and the absorbance of conjugates was measured at 280 and 780 nm (A280 and A780). The dye-to-antibody ratio and protein concentration were calculated using these absorbance values.
Purity of DTPA-trastuzumab-IRDye 800
The purity of DTPA-trastuzumab-IRDye 800 conjugates was determined by size-exclusion high performance liquid chromatography (SE-HPLC) (Hitachi High Technologies, Santa Clara, USA). The size-exclusion columns (TSK-Gel G3000SWXL) were pre-equilibrated with 0.05 mol/L phosphate buffer (pH 6.8), at a flow rate of 1 mL/min. The column eluent was evaluated using a diode array detector with monitoring at 280 and 780 nm.
Aliquots of 0.1-0.2 ng DTPA-trastuzumab-IRDye 800, mixed with Laemmli sample buffer (without beta-mercaptoethanol), were loaded onto 4-12% Tris-glycine acrylamide gels to evaluate purity by fluorescence. Gels were run until the bromophenol blue reached ~10 mm from the bottom of gels, and gels were imaged in an Odyssey NIR imaging system (Li-Cor, Lincoln, USA). The fluorescence intensity of free IRDye 800CW (about 1 kD) and trastuzumab conjugates (about 145 kD) were measured with ImageJ software to provide a percentage of the unbound IRDye 800, together with three-dimension (3D) surface plot.
Indium-111 labeling and radiochemical analysis
[111In]Cl3 was converted to [111In]-acetate by addition of 5 volumes of 0.1 mol/L sodium acetate (pH 7.0), and DTPA-trastuzumab-IRDye 800 was added at a ratio of 1.0 mg antibody to 10 mCi (370 MBq) of [111In]-acetate, and incubated for half an hour at room temperature. Prior to final purification, 10 µL of 20 mmol/L DTPA was added to the labeled preparation to quench free 111In. The Indium-111 radiolabeling yields were monitored using instant thin layer chromatography (ITLC) developed with 100 mmol/L sodium citrate as described above. [111In]-DTPA migrates with the solvent front (Rf =0.8-0.9), whereas dual-labeled trastuzumab conjugate remains at the application site (Rf =0-0.2).
Stability in buffer and human serum
Serum stability analysis was performed by adding 1 mCi of radiolabeled conjugates to 1 mL of PBS (pH 7.4) or human serum (Millipore). The mixtures were incubated at 37 °C for 24 h. Then, the samples were taken out and sodium dodecyl sulfate-polyacrylamide gel electrophoresis (SDS-PAGE) was performed. After electrophoresis, the gels were scanned with both a Bioscan imaging scanner and an Odyssey NIR imaging system. Images were analyzed by ImageJ software.
Immunoreactivity
The immunoreactivity of DTPA-trastuzumab-IRDye 800 was analyzed by flow cytometry before radiolabeling, and a binding assay was performed via Lindmo assay after labeling with [111In]Cl3. Human breast cancer SKBR-3 cells stably overexpressing HER2 were cultured as described previously.
Flow cytometry
The binding affinity of DTPA-trastuzumab-IRDye 800 was evaluated by flow cytometry, and compared with DTPA-trastuzumab. Briefly, 0.3×106 cells were incubated in 100 µL culture medium containing 5 µg of trastuzumab conjugates. After 30 min on ice, cells were washed once with 4 mL media, and then incubated for 30 min on ice with a fluorescein isothiocyanate (FITC)-conjugated mouse anti-human IgG antibody (BD Bioscience, USA) on ice. After a final wash, cells were resuspended in 2.5 µg/mL 7-Amino-actinomycin D. Mean fluorescence intensity (MFI) was assessed on a BD FACSAria. Trastuzumab, as a positive control, was tested at equivalent molar concentration, whereas an irrelevant polyclonal human immunoglobulin was set as a negative control.
Lindmo assay
A fixed amount of [111In]-DTPA-trastuzumab-IRDye 800 (100 ng) was added to increasing numbers of SKBR-3 cells (ranging from 0.1×106 to 2×106 in 0.5 mL). To determine non-specific cell binding, aliquots of cells were incubated with the radiolabeled conjugates in the presence of 50 µg unlabeled trastuzumab. After 1 h incubation, cellular binding was stopped by removal of the medium, followed by washing the cells with PBS (pH 7.4). The cell pellets were measured in a gamma counter. Specific binding was calculated as the ratio of cell-bound to total radioactivity applied minus nonspecific binding. The plot was set as the reciprocal of cell number (x-axis) vs. the reciprocal of specific binding rate (y-axis). The data were fitted with a least-squares linear regression method using OriginLabMicrocal software. The y-intercept gave the reciprocal of the immunoreactive fraction.
Internalization studies
SKBR-3 cells were seeded at a density of (0.8-1.1) ×106 cells/well in six-well plates and incubated overnight with internalization buffer (DMEM containing 1% fetal bovine serum) to obtain good cell adherence. The following day, SKBR-3 cells were pretreated with the internalization medium for 1 h at 37 °C. Approximately 2.5 pmol of [111In]-DTPA-trastuzumab-IRDye 800 was added to the medium, and the cells were incubated (in triplicate) for 4 and 24 h at 37 °C and 5% CO2. A 1,000-fold excess of each blocking agent was used to determine nonspecific internalization. At each time point, the internalization was stopped by removal of the medium followed by washing the cells with ice-cold 0.01 mol/L PBS (pH 7.4). Cells were then treated for 5 min (three times) with ice-cold glycine buffer (0.05 mol/L glycine solution, and pH was adjusted to 2.8 with 1 mol/L HCl) to distinguish between cell surface-bound (acid-releasable) and internalized (acid-resistant) radiolabeled antibody. The plates were then imaged using an Odyssey infrared imaging system. Finally, cells were detached from the plates by incubation with 1 mol/L NaOH for 10 min at 37 °C. The medium, the receptor-bound and the internalized fraction of [111In]-DTPA-trastuzumab-IRDye 800 were measured in a gamma counter, and the internalized radioactivity rate was calculated and normalized to 1×106 cells/well. The internalized fluorescence intensity was measured and plotted with ImageJ software.
In vivo biodistribution
The animal studies were approved from the Institutional Animal Care and Use Committee. Female nude BALB/c (nu/nu) mice (Harlan Laboratories), 4-5 weeks of age, were each subcutaneously (s.c.) implanted with 10 million SKBR-3 cells. When tumors grew to approximately 5 mm in diameter, nude mice were ready for in vivo study.
Nude mice were injected intravenously with 0.11 nmol (20 µg) of [111In]-DTPA-trastuzumab-IRDye 800 (about 1.48 MBq) in 0.1 mL of 0.9% NaCl solution via the lateral tail vein. For the determination of nonspecific uptake in tumor tissue, another group of 3 mice were pre-injected with 1 mg of trastuzumab dissolved in 0.1 mL of 0.9% NaCl solution 1 h prior to administration of the imaging agent. At 4, 24 and 48 h, the mice were anesthetized with isoflurane at a rate of 2.5% with 0.4 L/min oxygen through a non-rebreathing anesthesia vaporizer, and then killed with carbon dioxide gas. The organs of interest were collected, weighed, and radioactivity determined in a gamma counter together with injection standards. The percentage of injected dose per gram ( %ID/g) was calculated for each tissue. Tumor-to-tissue ratios were also determined.
Statistical analysis
Statistical analysis was processed using SPSS version 18.0 (SPSS Inc., Chicago, USA). Values are presented a . Student’s t-test was used to determine statistical significance. The significance level was set at 0.05.
Results
Preparation of dual-labeled imaging conjugate
On the average, 2.11±0.33 DTPA analogues were coupled to trastuzumab, and the conjugation reactions were performed at a 4:1 p-SCN-Bn-DTPA-to-trastuzumab ratio; the dye-to-protein ratio of DTPA-trastuzumab-IRDye 800 was calculated as 1.92±0.26 using a 4-fold molar excess of IRDye 800CW. The radiolabeling yield of [111In]-DTPA-trastuzumab-IRDye 800 was >90% at a specific activity of 13.7-137.0 MBq/µmol, as demonstrated by ITLC analysis.
Purity analysis of DTPA-trastuzumab-IRDye 800
Chemical and optical purities of DTPA-trastuzumab-IRDye 800 were evaluated via SE-HPLC and SDS-PAGE (Figure 2). Figure 2A,B show the retention time of bioconjugate to be 8.80 min at 280 and 780 nm, compared to 9.26 min for trastuzumab and 12.39 min for p-SCN-Bn-DTPA at 280 nm and 12.51 min for IRDye 800CW at 780 nm. The chromatogram of DTPA-trastuzumab-IRDye 800 revealed no peak representing free DTPA or unconjugated IRDye 800.
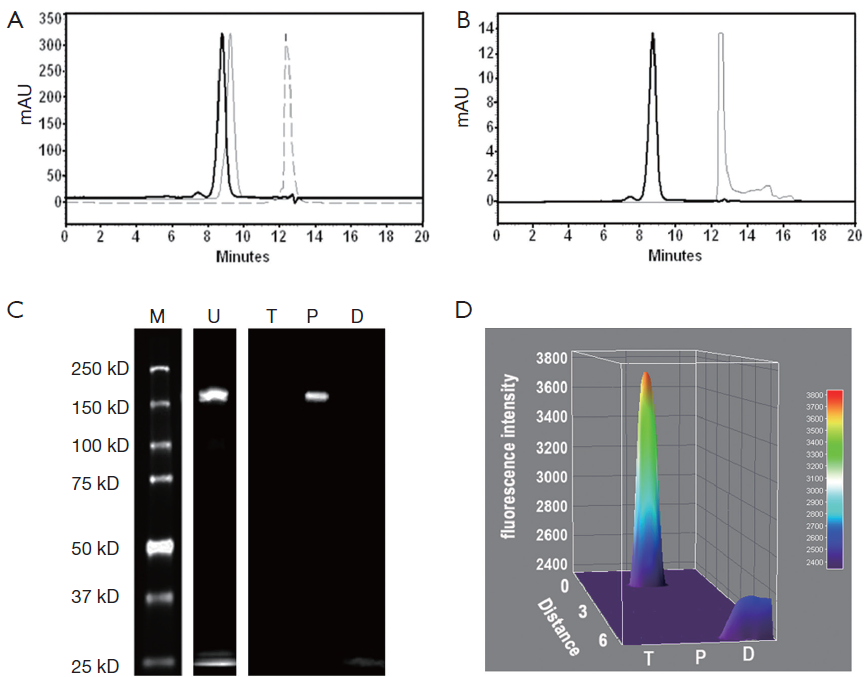
SDS-PAGE and fluorescence imaging were used as an optimal method of determining the optical purity of DTPA-trastuzumab-IRDye 800 (Figure 2C,D). The location of free IRDye 800CW in the gel (about 1 kD), was similar to that of bromophenol blue (about 500 Da). In the unpurified conjugate, a free IRDye 800CW band was displayed in addition to about 150 kD band (trastuzumab conjugates). After the purification of bioconjugate, no free dye band or fluorescence peak was detected from the gel or the 3D surface plot.
Immunoreactivity of DTPA-trastuzumab-IRDye 800
Figure 3A illustrated flow cytometry results from SKBR-3 cells incubated with DTPA-trastuzumab-IRDye 800. The histogram of DTPA-trastuzumab showed no reduction of fluorescent intensity, suggesting an intact binding affinity compared to trastuzumab. The binding capacity of DTPA-trastuzumab-IRDye 800 was mildly impaired, as demonstrated by a shift of the histogram towards the histogram of SKBR-3 cells not incubated with the conjugate.
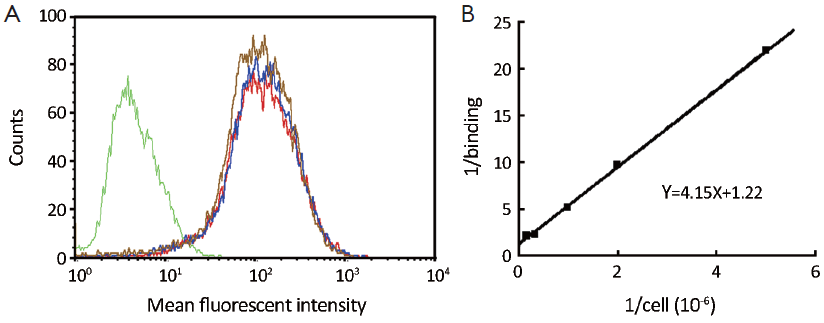
The Lindmo assay was used to determine immunoreactivity fraction of [111In]-DTPA-trastuzumab-IRDye 800 (Figure 3B). A linear relationship between TA/SB and 1/(cells) was established. The best fraction of immunoreactivity was calculated as r=1/1.22=0.82 (Y=4.15X+1.22). The average fraction of immunoreactivity was 0.78±0.04 (n=5).
Stabilities in buffer and human serum
Optical stability of [111In]-DTPA-trastuzumab-IRDye 800 in PBS or human serum was demonstrated using a Li-Cor Odyssey near infrared imaging system. Figure 4A illustrated that dual-labeled conjugate was stable in PBS after 24 h, as determined by PAGE. When the dual-labeled conjugate was incubated with human serum for 24 h, (8.43±0.71)% of the total bioconjugate was found to form into larger proteins (>150 kD) and extra bands were detected on the SDS. Fluorescence intensities of each component were measured and illustrated as a 3D surface plot. [111In]-DTPA-trastuzumab-IRDye 800 without any incubation was used as a reference, and only a single fluorescent peak was seen on the plot (Figure 4B). The retention time of dual-labeled immunoconjugate incubated in PBS remained the same as the reference conjugate, whereas two extra fluorescence peaks corresponding to larger molecular weight proteins were seen in the serum stability study (Figure 4C).
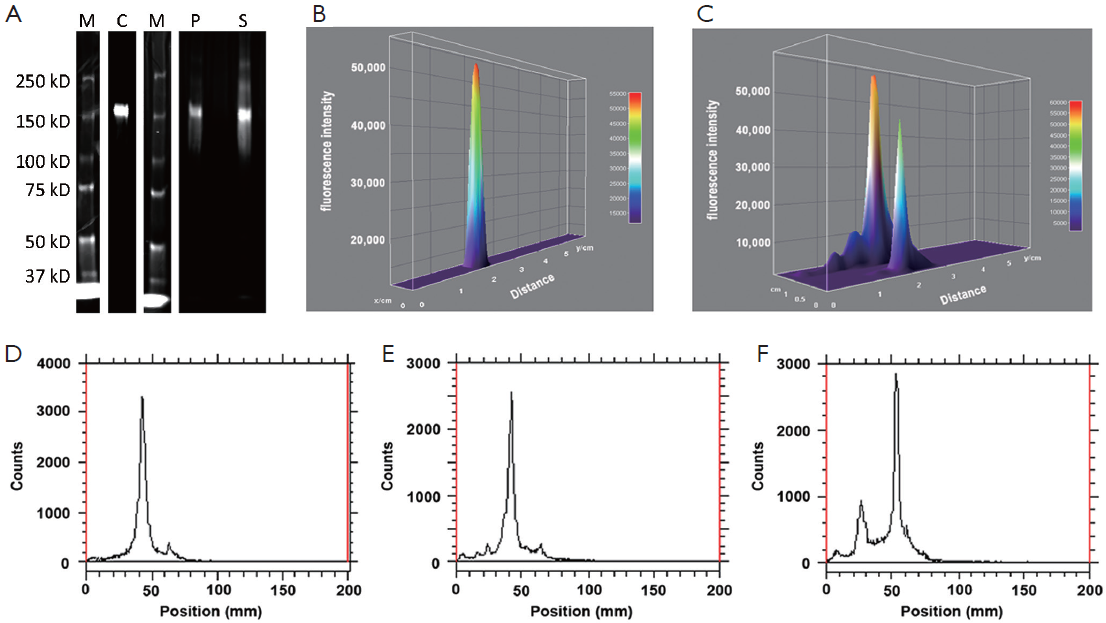
Similar results were obtained after SDS-PAGE gels were scanned with Bioscan TLC imaging (Figure 4D-F). Compared with the histogram of [111In]-DTPA-trastuzumab- IRDye 800 at 0 h, an extra peak corresponding to higher molecular proteins was seen in the serum stability plot at 24 h (Figure 4F). The PBS stability profile at 24 h was the same as the profile at 0 h (Figure 4E). These results suggested that nuclear and optical purity are highly correlated.
Internalization study
The internalization property of [111In]-DTPA-trastuzumab-IRDye 800 was studied in SKBR-3 cells at 37 °C in the absence or presence of a 1,000-fold excess of trastuzumab (nonspecific uptake). [111In]-DTPA-trastuzumab-IRDye 800 showed specific cell uptake at 37 °C. The amount of internalized radioactivity was (22.95±1.56)% at 4 h, and the value increased to (29.17±3.08)% at 24 h. The total fluorescence intensity associated with internalized IRDye 800 at 4 and 24 h is shown in Figure 5. The fluorescence intensity from the surface plot for 24 h also showed an increase in over 4 h.
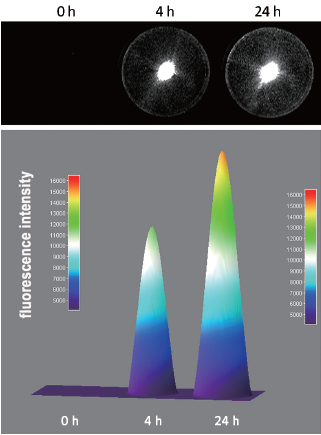
Biodistribution study
Biodistribution of [111In]-DTPA-trastuzumab-IRDye 800 was assessed in SKBR-3 tumor-bearing nude mice at 4, 24 and 48 h, and all data at each time point are summarized in Table 1 as %ID/g tissue.
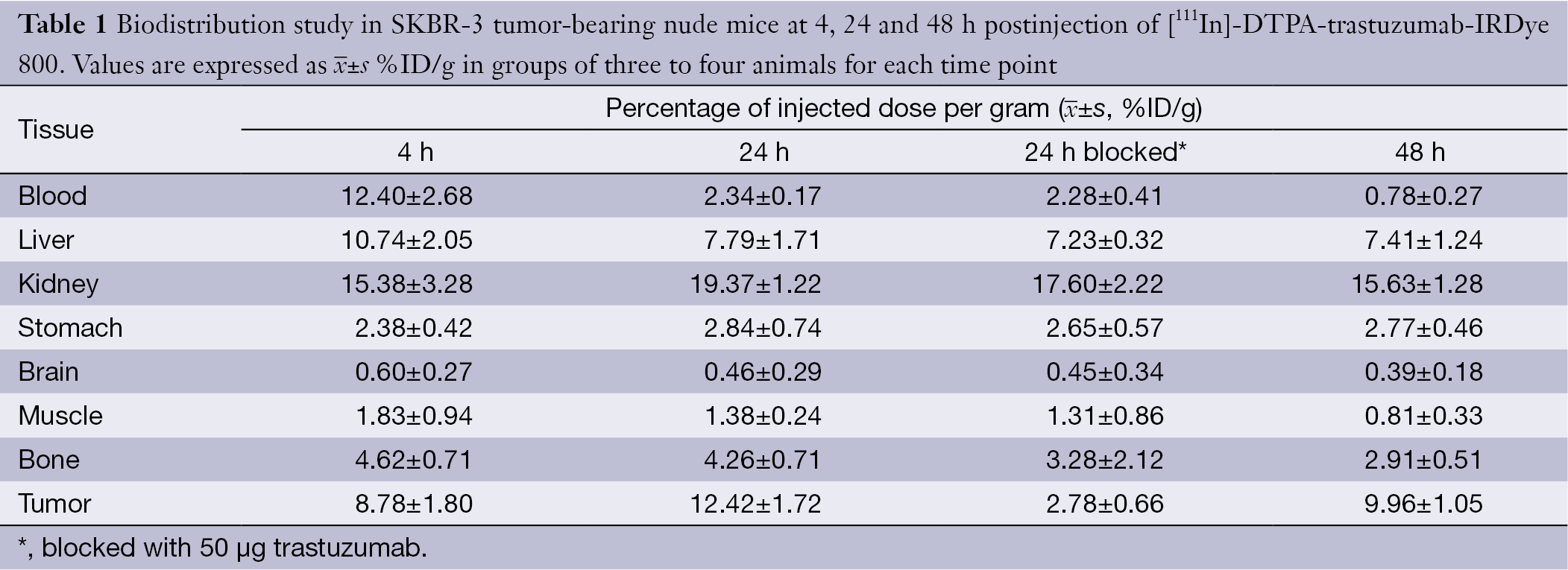

Full table
[111In]-DTPA-trastuzumab-IRDye 800 displayed rapid blood clearance from SKBR-3-bearing mice. The radioactivity in the blood was 12.40±2.68 %ID/g at 4 h and 2.34±0.17 %ID/g at 24 h. After 48 h, only 1.22±0.66 %ID/g of radioactivity was seen in the blood. When animals with HER2-expressing tumors were given [111In]-DTPA-trastuzumab-IRDye 800, relatively high uptake was observed in HER2-expressing tumors (8.78±1.80 %ID/g) 4 h postinjection of the dual-labeled conjugate. Tumor accumulation increased to 12.42±1.72 %ID/g at 24 h, and 9.96±1.05 %ID/g of [111In]-DTPA-trastuzumab-IRDye 800 remained in the SKBR-3 tumor at 72 h. The uptake is specific for HER2-positive tumors, as indicated by the 78% loss of tumor retention at 24 h with pre-injection of unlabeled trastuzumab. The tumor-to-tissue-ratio is an important parameter in evaluating the specificity of a targeting agent. The tumor-to-muscle ratios of [111In]-DTPA-trastuzumab-IRDye 800 were 9.13±1.68 at 24 h and 12.79±2.13 at 48 h.
Excretion of the dual-labeled conjugate was mainly via the kidney and liver. The kidney uptake was 19.37±1.22 %ID/g at 24 h, decreasing to 15.63±1.28 %ID/g at 48 h; whereas the liver uptake was 7.41±1.24 %ID/g at 48 h postinjection.
Discussion
Use of a dual-labeled molecular imaging agent for imaging and therapy is promising, because this technique can provide an opportunity for imaging and fluorescence-guided resection without a concern for fluorophore half-life (13,14). Other than small-animal studies, the fusion of nuclear and optical methods is a less frequently used multimodality imaging strategy. This infrequent use can be attributed to a variety of reasons, one of which is the lack of US Food and Drug Administration (FDA)-approved target-specific diagnostic imaging agents.
Our previous work with a targeted dual-labeled trastuzumab-based imaging agent did not have a tumor-to-tissue ratio high enough for further translation (3). Thus, the primary objective of this study was to optimize and standardize the preparation procedure in order to modify the antibody as much as possible while insuring that bioreactivity was not compromised.
Many radioactive reporters have demonstrated the possibility of dimer or multimer formation when linking more DTPA or fluorophore to an antibody, with a resulting decrease in biological affinity of the conjugate relative to the unlabeled antibody (12). So we decreased the DTPA-to-protein ratio of the dual-labeled trastuzumab to increase immunoreactivity while not adversely affecting the radiolabeling yield. To optimize the “m” number, we synthesized a serial of NIR-labeled trastuzumab. Flow cytometry data showed increasing dye-to-antibody ratios decreased the immunoreactivity of antibody-based conjugate (in press). Furthermore, other data revealed that the NIR dye has greater physical-chemical interference with molecular components than chelator does; so optimal dye-to-protein ratio can be very critical to near infrared, molecular fluorescence imaging.
In this study, we synthesized a dual-labeled trastuzumab-based imaging agent, [111In]-(DTPA)2.11-trastuzumab-(IRDye 800)1.92. Results of flow cytometry and the Limdo assay showed that most of immunoreactivity of the dual-labeled conjugate was preserved. The cellular retention of [111In]-(DTPA)2.11-trastuzumab-(IRDye 800)1.92 was prolonged, which explained why radioactivity was slowly released from HER2-positive tumors in animal studies. The biodistribution study in mice illustrated the targeting potential of dual-labeled trastuzumab with a tumor overexpressing the HER2 receptor. Relatively high tumor uptake was observed at all time points, and the maximum uptake was found to be 12.42±1.72 %ID/g 24 h after injection of the dual-labeled conjugate. The tumor-to-muscle ratios of [111In]-(DTPA)2.11-trastuzumab-(IRDye 800)1.92 were 9.13±1.68 at 24 h and 12.79±2.13 at 48 h, compared to 2.25 at 24 h and 2.5 at 48 h for [111In]-(DTPA)10-trastuzumab-(IRDye 800)7-10 as reported in previous work (4).
Apart from the work described above, we also concentrated on developing and validating analytical approaches to assess purity, stability, and immunoreactivity of dual-labeled imaging agents prior to human administration.
The greatest concern in this study was optical purity and stability of the dual-labeled imaging agent, because free dye can create a significant background that can limit the performance of an optical molecular imaging. We previously reported a simple and rapid method for determining purity using polyacrylamide gel separation to quantify free/unconjugated dye in dual-labeled conjugates, and addressed validation parameters of accuracy and precision, as well as limits of detection and quantification (15). We found that extremely low amounts of fluorophore could be detected in conjugate via this assay while HPLC assessment of free dye was not possible. Furthermore, the gel electrophoresis and radioactive imaging system was validated as a good choice for evaluating the radiostability of the dual-labeled antibody-based agents.
Even though Lindmo assay is acknowledged as a standard method to determine the immunoreactivity of radiolabeled antibody-based agent, it has several shortcomings that cannot be validated for the Good Manufactory Practice, for example, compensating for a lower affinity to produce a higher immunoreactivity, tedious preparation of large numbers of cells, wasting amount of unlabeled antibody, and so on. Our future strategy is to use the flow cytometry with appropriate excitation and emission wavelengths to directly excite the NIR fluorophore to directly evaluate the immunoreactivity of dual-labeled compounds.
Conclusions
Although studies of toxicity and dosimetry remain to be performed, optimizing the preparation method of the dual-labeled trastuzumab-based imaging agent significantly improves immunoreactivity and thus tumor targeting, and moves this imaging agent much closer to clinical application.
Acknowledgements
Funding: This work was supported by Beijing Natural Science Foundation (No. 7132037) and NIH R01.
Disclosure: The authors declare no conflict of interest.
References
- Wu AM, Olafsen T. Antibodies for molecular imaging of cancer. Cancer J 2008;14:191-7. [PubMed]
- Sampath L, Kwon S, Hall MA, et al. Detection of Cancer Metastases with a Dual-labeled Near-Infrared/Positron Emission Tomography Imaging Agent. Transl Oncol 2010;3:307-217. [PubMed]
- Sampath L, Kwon S, Ke S, et al. Dual-labeled trastuzumab-based imaging agent for the detection of human epidermal growth factor receptor 2 overexpression in breast cancer. J Nucl Med 2007;48:1501-10. [PubMed]
- Corcoran EB, Hanson RN. Imaging EGFR and HER2 by PET and SPECT: a review. Med Res Rev 2014;34:596-643. [PubMed]
- Carter P, Presta L, Gorman CM, et al. Humanization of an anti-p185HER2 antibody for human cancer therapy. Proc Natl Acad Sci U S A 1992;89:4285-9. [PubMed]
- Hudis CA. Trastuzumab--mechanism of action and use in clinical practice. N Engl J Med 2007;357:39-51. [PubMed]
- Slamon DJ, Godolphin W, Jones LA, et al. Studies of the HER-2/neu proto-oncogene in human breast and ovarian cancer. Science 1989;244:707-12. [PubMed]
- Maeda A, Bu J, Chen J, et al. Dual In Vivo Photoacoustic and Fluorescence Imaging of HER2 Expression in Breast Tumors for Diagnosis, Margin Assessment, and Surgical Guidance. Mol Imaging 2014;13:1-9. [PubMed]
- Lub-de Hooge MN, Kosterink JG, Perik PJ. Preclinical characterisation of 111In-DTPA-trastuzumab. Br J Pharmacol 2004;143:99-106. [PubMed]
- Tamura K, Kurihara H, Yonemori K, et al. 64Cu-DOTA-trastuzumab PET imaging in patients with HER2-positive breast cancer. J Nucl Med 2013;54:1869-75. [PubMed]
- Goldstein R, Sosabowski J, Vigor K, et al. Developments in single photon emission computed tomography and PET-based HER2 molecular imaging for breast cancer. Expert Rev Anticancer Ther 2013;13:359-73. [PubMed]
- Hnatowich DJ, Childs RL, Lanteigne D, et al. The preparation of DTPA-coupled antibodies radiolabeled with metallic radionuclides: an improved method. J Immunol Methods 1983;65:147-57. [PubMed]
- Sevick-Muraca EM, Rasmussen JC. Molecular imaging with optics: primer and case for near-infrared fluorescence techniques in personalized medicine. J Biomed Opt 2008;13:041303. [PubMed]
- Sevick-Muraca EM, Sharma R, Rasmussen JC, et al. Imaging of lymph flow in breast cancer patients after microdose administration of a near-infrared fluorophore: feasibility study. Radiology 2008;246:734-41. [PubMed]
- Aldrich MB, Wang X, Hart A, et al. Assessment of free dye in solutions of dual-labeled antibody conjugates for in vivo molecular imaging. Mol Imaging Biol 2011;13:32-42. [PubMed]