IGHG1 promotes motility likely through epithelial-mesenchymal transition in ovarian cancer
Introduction
Ovarian cancer (OC) ranks as the fifth most common cause of mortality for female cancer patients, accounting for 151,900 deaths worldwide (1,2). The incidence of this devastating malignancy has been rising currently (3-5). The majority of OC patients are diagnosed at advanced stages of disease. Although therapeutics has been developed to improve treatment outcome, prognosis of the OC patients at late stage remains unfavorable (6). Only one out of four patients with advanced OC could survive for five years after diagnosis (2). One reason for the gloomy survival rate is rapid development of chemo-resistance. For example, 25% of OC patients become unresponsive to platinum drugs within 6 months after the surgery and initial chemotherapy (7). Another reason is metastasis of OC cells, which rules out surgery and potentially neutralizes traditional chemotherapy strategies (8,9). Therefore, it is critical to uncover molecular mechanisms underlying the two fatal symptoms in OC and develop novel therapeutic methods accordingly.
Identifying unique antigens in malignancy and developing specific antibodies accordingly provide a critical therapeutical option (10). In the late 1980s, the antibody COC166-9 was found to be highly specific in OC (11), and experimental therapy showed that COC166-9 conjugated to cisplatin or adriamycin significantly inhibits xenograft tumor growth in immunocompromised mice (12). Further investigation revealed that immunoglobulin γ-1 heavy chain constant region (IGHG1) is the antigen target of COC166-9 and correlates with poor prognosis and recurrence in OC patients (13). However, the function of IGHG1 in OC progression remains poorly understood.
In this study, we found that IGHG1 expression is higher in OC samples compared with the adjacent normal counterparts. Additionally, increased IGHG1 level is associated with lymph node metastasis. IGHG1 promotes migration and invasion in OC cells likely through modulating epithelial-mesenchymal transition (EMT) program, without affecting proliferation and apoptosis. Together, these results enlighten the roles of IGHG1 in OC progression and provide more evidence for applying COC166-9 in clinical practice.
Materials and methods
Cell culture and reagents
OC cell line Caov-3 and SKOV3, including 3AO, were purchased from the China Military Science Center (Beijing, China). OC3 cells (14) were generously provided by Dr. Hongxia Li (Department of Obstetrics and Gynecology, Beijing Shijitan Hospital of Capital Medical University, China). COC1 and COC1/DDP cells were gifted from Peking Union Medical College Hospital. The ovarian carcinoma cell line HOC1A, derived from poorly differentiated OC surgical specimens, was cultured and preserved in Center of Gynecological Oncology, Department of Obstetrics and Gynecology, Peking University People’s Hospital. Caov-3 cells were cultured in dulbecco’s modified eagle medium (DMEM) supplemented with 10% fetal calf serum (FBS), 100 U/mL ampicillin, and 100 mg/mL streptomycin. All the other OC cell lines including two derived sublines (SKOV3.ip and COC1/DDP) were cultured in RPMI1640 containing 10% FBS, 100 U/mL ampicillin, and 100 mg/mL streptomycin. All cells were maintained under the humid conditions (37°C, 5% CO2).
Primary antibodies against E-cadherin (sc-7870), N-cadherin (sc-7939), vimentin (sc-32322), and glyceraldehyde-3-phosphate dehydrogenase (GAPDH) (sc-59540) were purchased from Santa Cruz Biotechnology (Dallas, TX, USA). The Cleaved Caspase-3 (Asp175) antibody (9661) was purchased from Cell Signaling Technology (MA, USA). An anti-IGHG1 antibody was generated and characterized in Center of Gynecological Oncology, Department of Obstetrics and Gynecology, Peking University People’s Hospital. Three specific siRNAs against IGHG1 were purchased from Invitrogen (Carlsbad, CA, USA). 3-(4,5-dimethylthiazol-2-yl)-2,5-diphenyltetrazolium bromide (MTT) and propidium iodide (PI) were purchased from Sigma-Aldrich (St. Louis, Missouri, USA).
RNA and real-time quantitative polymerase chain reaction (RT-qPCR)
Total RNA was extracted from cells using the standard Trizol (Invitrogen) procedure. RNA quality was assessed and treated with a TURBO DNA-free Kit (Invitrogen). SuperScript III (Invitrogen) was used to prepare cDNA. Quantitative PCR was performed using the TransScript II Green One-Step RT-qPCR SuperMix (TransGen Biotech, Beijing, China). Primers used to detect IGHG1 were: 5’-GCAGCCGGAGAACAACTACA-3’ (forward) and 5’-TGGTTGTGCAGAGCCTCATG-3’ (reverse). Primers for the GAPDH internal control were: 5’-TGTTGCCATCAATGACCCCTT-3’ (forward) and 5’-CTCCACGACGTACTCAGCG-3’ (reverse). The abundance of IGHG1 mRNA was evaluated using the 2–ΔΔCt method.
Plasmids, RNAi and transfection
IGHG1 was cloned into pcDNA3.1 (pcDNA3.1-IGHG1) and the construct was verified by sequencing (Invitrogen). Plasmids or a pool of three siRNAs (100 nmol/L in total) were transiently transfected into OC cells using ScreenFect A (Incella, Eggenstein-Leopoldshafen, Germany) according to the manufacturer’s instructions.
Proliferation assay
MTT assay and flow cytometry were used to detect the viability of OC cells. The same number of cells (6×103 cells/well) was plated into 96-well dish and OD490 was detected in three consecutive days. As for cell cycle assay, cells were harvested using trypsin and fixed using 75% ethanol. PI was added into the suspended cells before analysis. MTT assays and flow cytometry were carried out twice independently and five biological replicates were involved in each assay.
Apoptosis assay
We examined apoptosis in Caov-3 or OC3 cells using the FITC Annexin V/Dead Cell Apoptosis Kit (Invitrogen), according to the manufacturer’s instructions. The Annexin V-PI assay was performed twice independently and three biological replicates were involved in each assay. The levels of cleaved-Caspase3 in IGHG1-manipulated Caov-3 and OC-3 cells were detected by western blotting.
Transwell assay
Cellular migration and invasion in vitro were tested as previously described (15). In invasion assay, 100 μL FBS-free DMEM or RPMI1640 containing 3.6 μL Matrigel (BD, Franklin Lakes, New Jersey, USA) was added into the inserts. After 2 h, 1×105 cells were seeded into each insert and incubated for 8 h. The inserts were then harvested, fixed and stained with crystal violet. Five fields were selected randomly and cells that had penetrated the membrane were counted. Data are shown as x±s.
Immunoblot assay
Proteins were extracted from cells with lysis buffer (100 mmol/L NaCl, 10 mmol/L EDTA (pH 8.0), 50 mmol/L Tris-Cl (pH 8.0) and 0.5% (v/v) Triton X-100) containing protease inhibitor (Roche, Basel, Switzerland). Protein concentration was determined using BCA method (Thermo Fisher Scientific, MA, USA). Total proteins were separated on SDS-PAGE gel and transferred to PVDF membranes. GAPDH was used as the loading control.
Immunohistochemistry (IHC)
IHC assay was performed as previously described (16). All experiments on OC samples were approved by the Institute Research Medical Ethics Committee of Peking University People’s Hospital. Results were analyzed independently by two pathologists from the Department of Pathology in Peking University People’s Hospital (Beijing, China).
Statistical analysis
Except the IHC assay, all experiments were performed independently twice at least. Two-tailed Student’s t-test or Chi-square test was used to analyze the significance of data. Data are presented as x±s. GraphPad Prism 6 (GraphPad Software, Inc., CA, USA) was used to draw bar charts. In bar graphs, *, ** and *** indicate P<0.05, P<0.01 and P<0.001, respectively.
Results
IGHG1 expression in OC specimens and cell lines
First, we examined IGHG1 expression in 94 pairs of OC and adjacent normal tissues, showing that IGHG1 expressions in malignant tissues were dramatically increased compared with the adjacent normal counterparts (Figure 1A). Chi-square test was utilized to compare the differences in IGHG1 protein expression between OC tissues and adjacent noncancerous tissues (P<0.05). Further analysis revealed that the elevated IGHG1 expression correlated with the lymph node metastasis of OC (P=0.04,Figure 1B), implying that IGHG1 probably involves in regulating motility of OC cells. Then we tested the endogenous expression of IGHG1 in a panel of OC cell lines using quantitative PCR, among which three cell lines (Caov-3, SKOV3, and SKOV3.ip) possessed higher IGHG1 levels than the rest ones (COC1, COC1/DDP, ES-2, OC3, and 3AO) (Figure 1C).
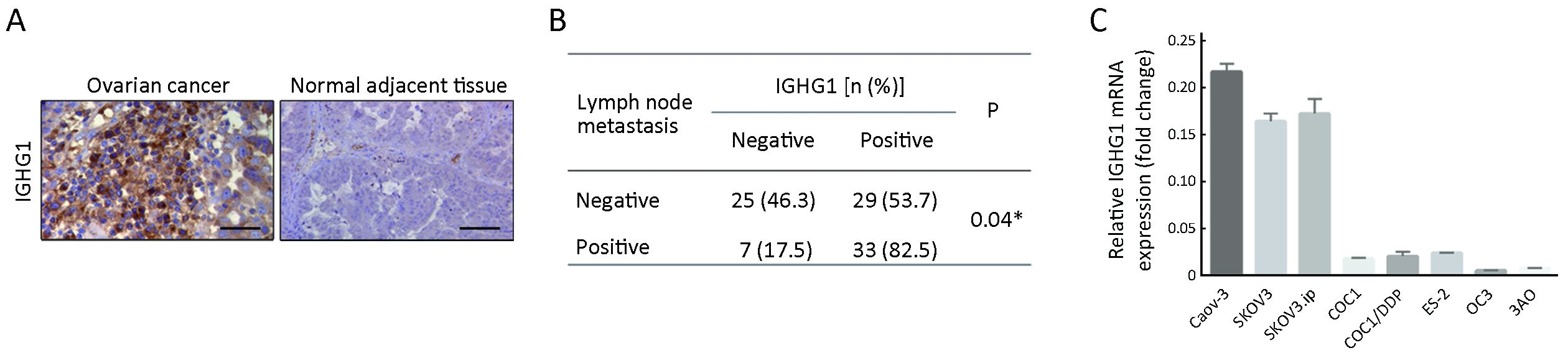
Two cell lines with high IGHG1 expressions (Caov-3 and SKOV3) and two with low IGHG1 levels (OC3 and 3AO) were used for analyzing the functions of IGHG1 in OC. We introduced a pool of three specific siRNAs against IGHG1 into Caov-3 and SKOV3 cells respectively to silence IGHG1 expressions significantly (Figure 2A, B). We also transfected plasmid pcDNA3.1-IGHG1 and the control plasmid into OC3 and 3AO cells respectively, and the increased IGHG1 levels were then confirmed by using quantitative PCR and immunoblot assay (Figure 2C, D).
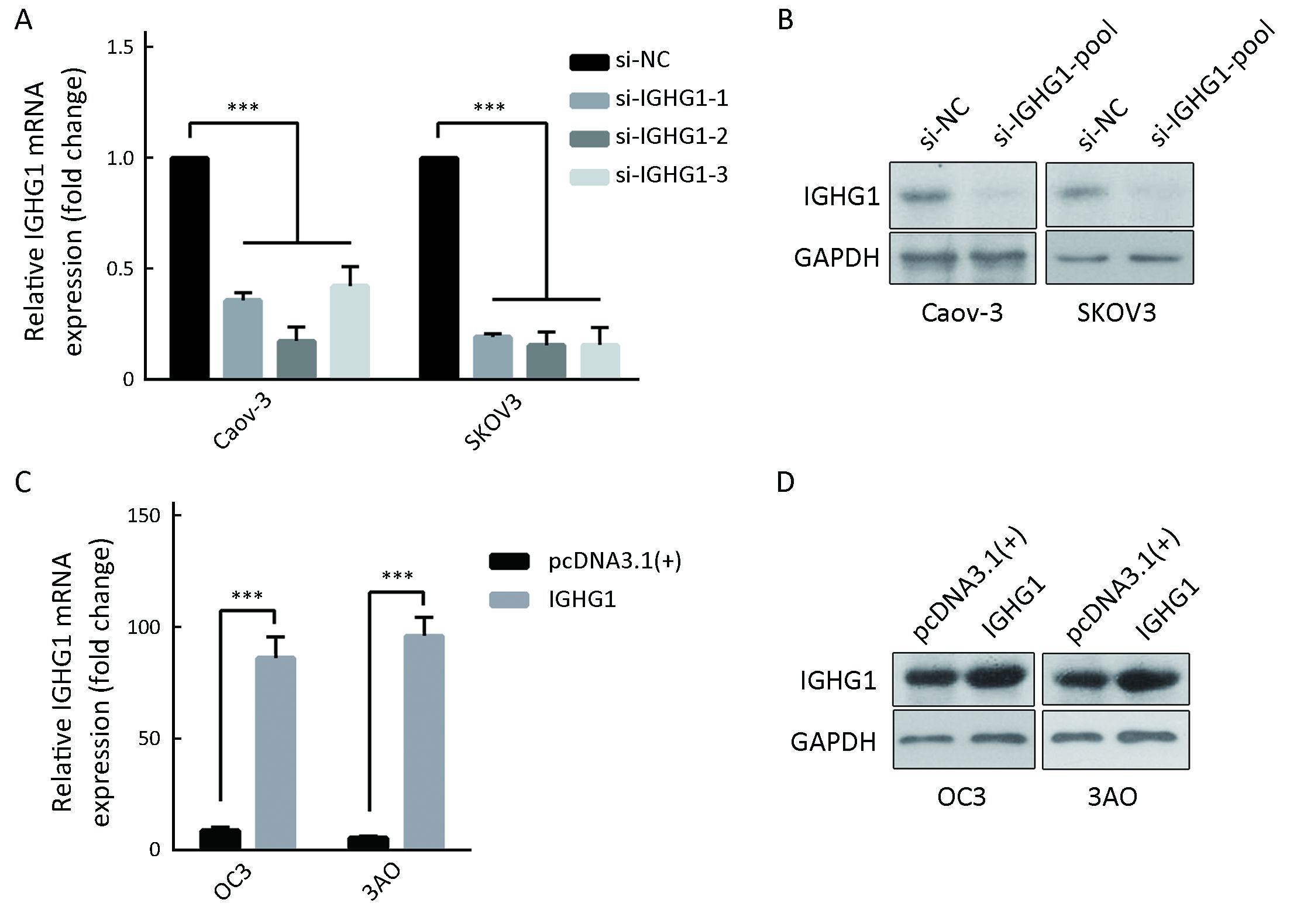
IGHG1 does not affect the proliferation of OC cells
To test whether IGHG1 would affect the proliferation of OC cells or not, we firstly used MTT assay to detect the viability of OC cells within three days. MTT assays showed that decreased expressions of IGHG1 in Caov-3 and SKOV3 cells did not change the proliferation in these treated cells compared with the control ones. Likewise, elevated IGHG1 level failed to influence the growth of OC3 cells as well. The results are reported as x±s, and statistical significance was assessed by two-tailed Student’s t tests with the level of significance set at P<0.05. The experiments were performed twice independently with five biological replicates. Increased or decreased expression of IGHG1 did not influence growth of the manipulated cells compared with those control cells (P>0.05) (Figure 3A). Furthermore, silence of IGHG1 in both Caov-3 and SKOV3 cells failed to alter the cell cycle distribution (P>0.05) (Figure 3B).
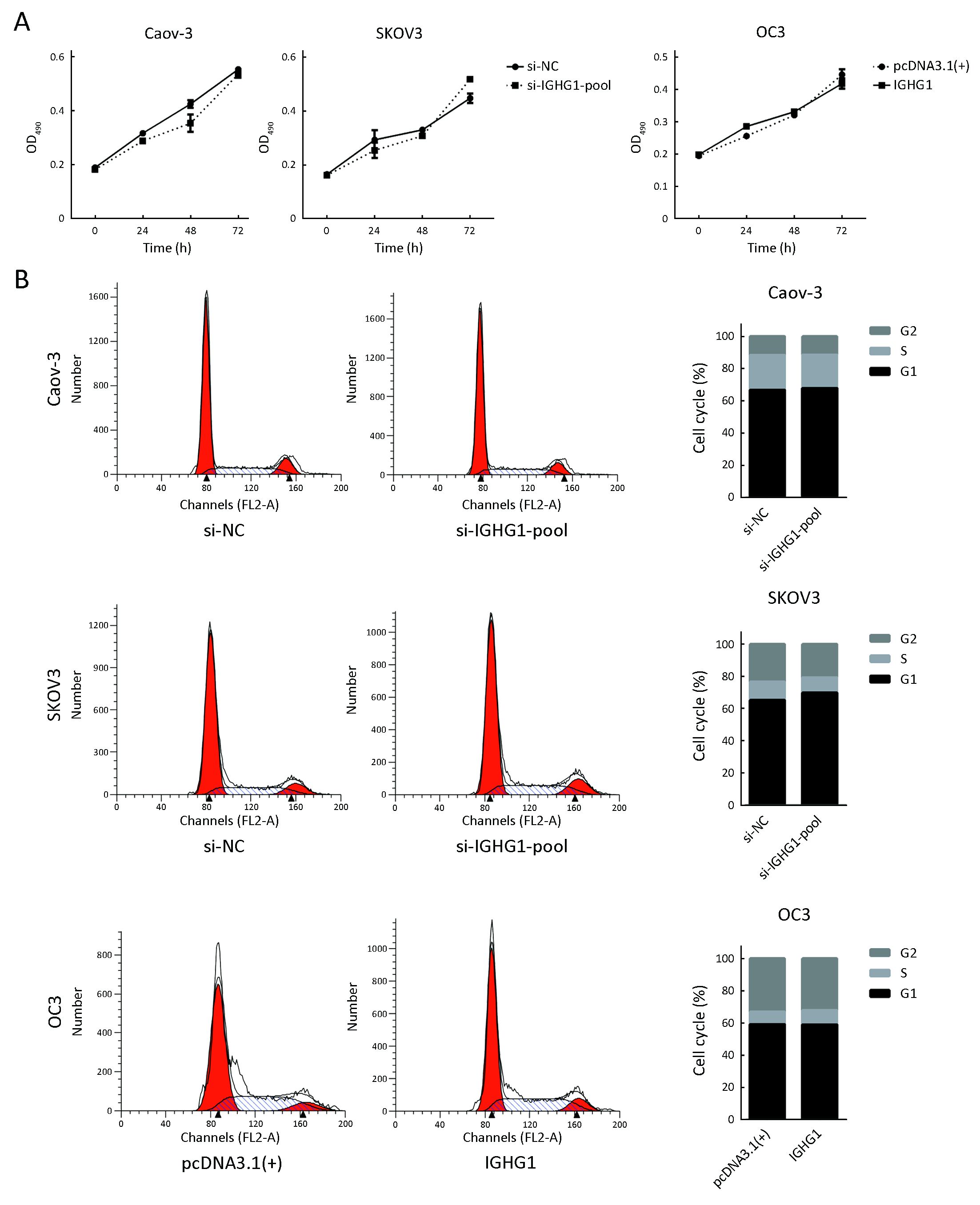
IGHG1 does not influence the apoptosis of OC cells
In order to test the potential effect of IGHG1 on apoptosis, we detected the apoptosis rate in OC cells using PI & Annexin V assay. The assay was performed twice independently and three biological replicates were involved in each assay. Neither silence of IGHG1 in Caov-3 cells nor overexpression of IGHG1 in OC3 cells influences the apoptosis in these manipulated cells, comparing with the control counterparts (P>0.05). Figure 4 shows one representative result. Knockdown of IGHG1 in Caov-3 cells exerted little effect on apoptosis (Figure 4A). Likewise, the increased IGHG1 levels in OC3 cells neither promoted nor inhibited apoptosis relative to those control counterparts (Figure 4B). The levels of cleaved caspase 3 in Caov-3 and OC-3 cells were detected by western blotting, and no alterations were observed between the control and IGHG1-manipulated cells (Figure 4C, D). The experiments were performed twice independently.
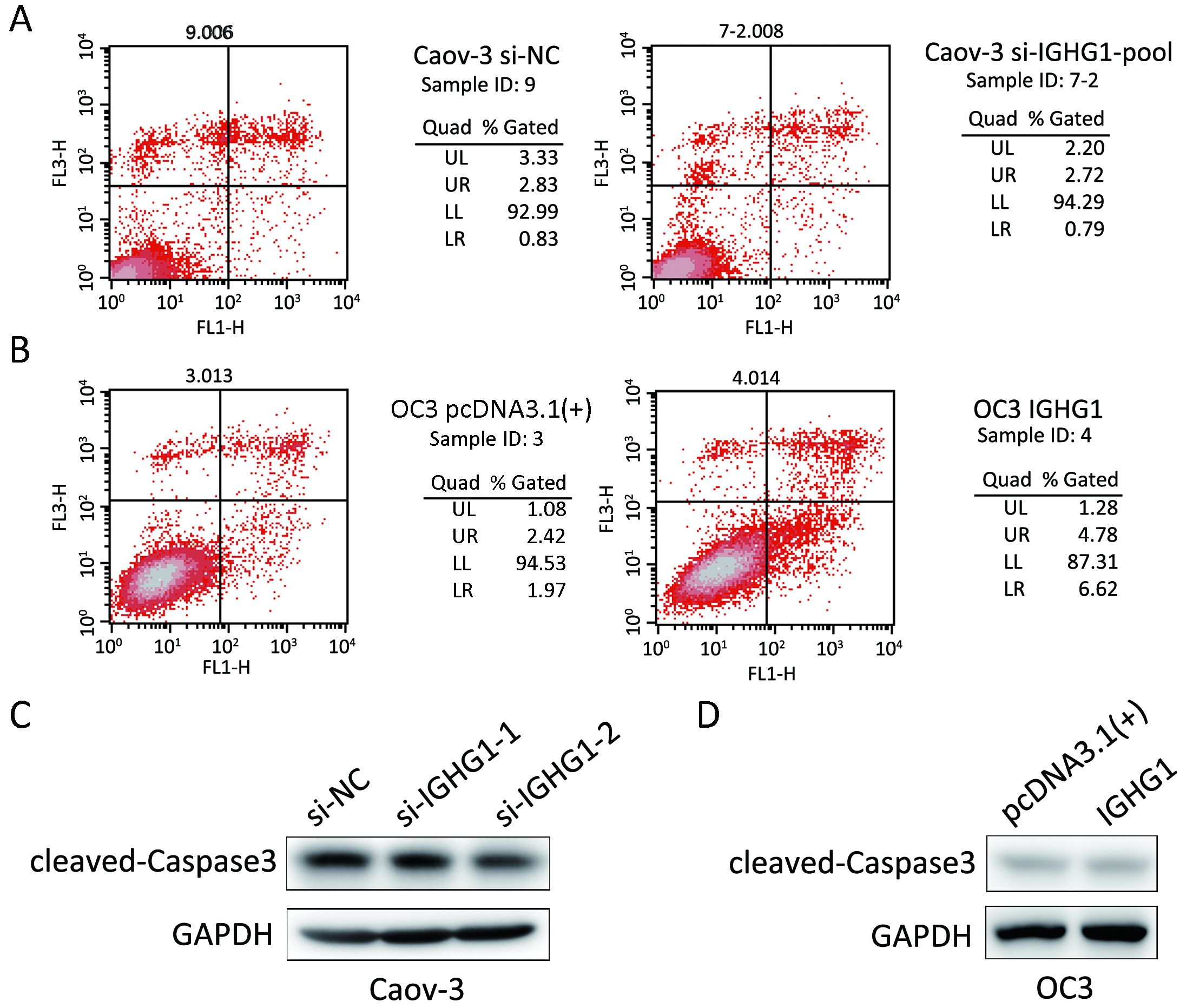
IGHG1 promotes motility of OC cells
Since IGHG1 expression is associated with the lymph node metastasis, then we examined whether IGHG1 promoted the motility of OC cells or not. We found that decreased IGHG1 expression in SKOV3 cells impaired in vitro migration and invasion relative to the control cells (Figure 5A). On the other side, overexpression of IGHG1 stimulated more 3AO cells to penetrate the transwell membrane in both migration and invasion assays (Figure 5B).
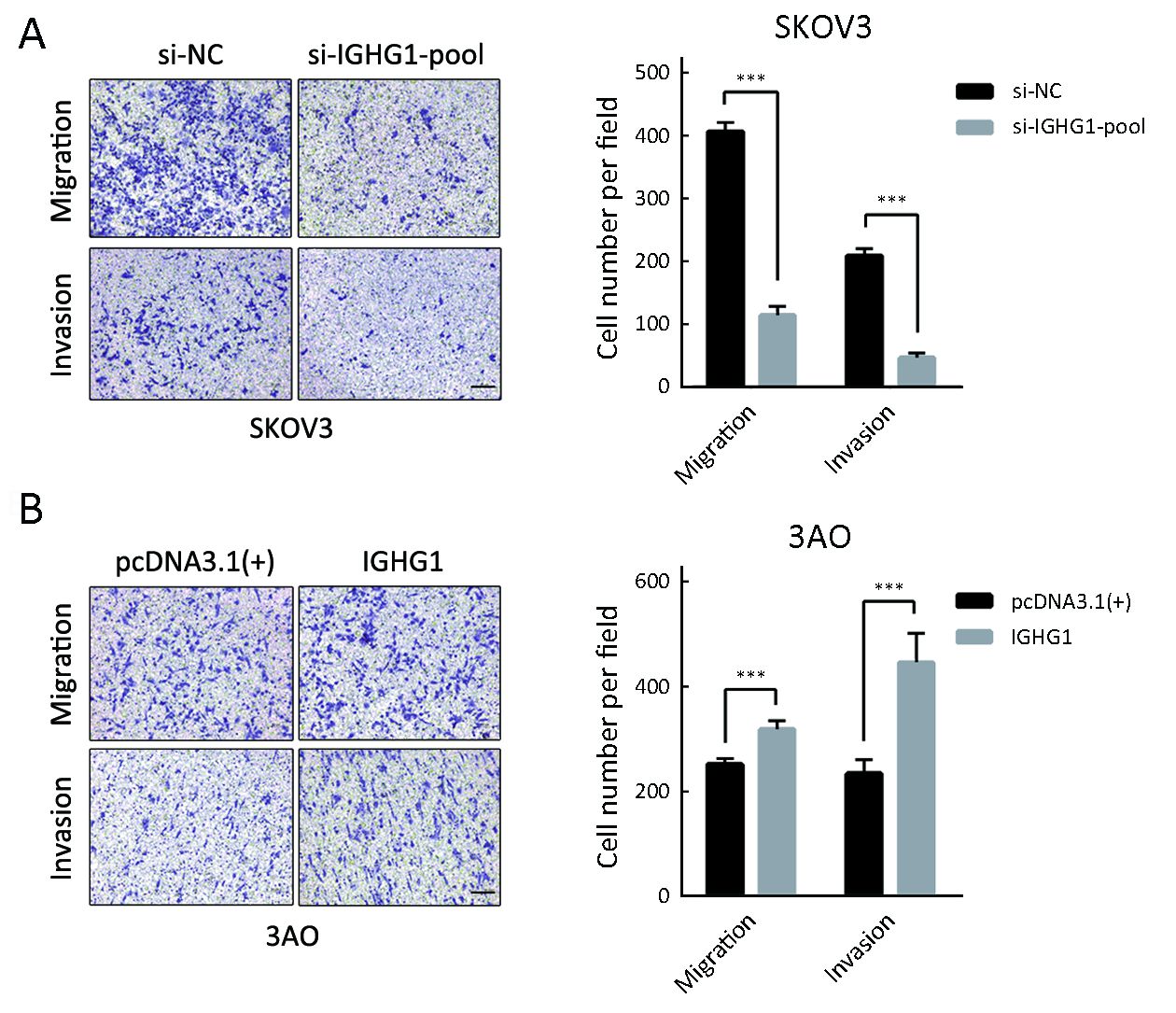
As EMT program underlies the enhanced motility and metastasis of OC cells (17), we then examined whether or not IGHG1 promoted motility of OC cells through modulating EMT. As expected, E-cadherin level increased while N-cadherin and Vimentin decreased in SKOV3 cells owing to silencing of IGHG1 (Figure 6A). Vice versa, overexpression of IGHG1 in 3AO cells promoted the expression of N-cadherin and Vimentin while inhibited E-cadherin expression (Figure 6B). Collectively, these data demonstrate that IGHG1 enhances the motility of OC cells likely through EMT.
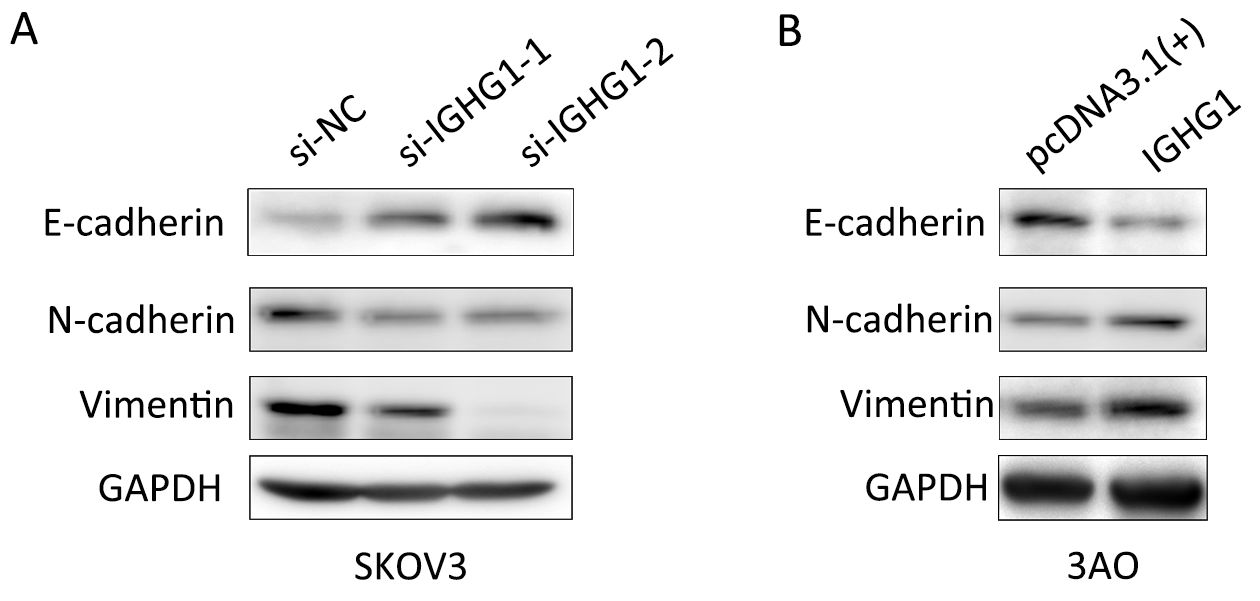
Discussion
IGHG1 has recently been determined as the antigen of the antibody COC166-9 and aberrantly increased expression of IGHG1 correlates with poor prognosis in OC patients (13). We thereby set out to explore the function of IGHG1 in OC progression. Ji et al. reported that IGHG1 promotes the proliferation of HOC1A cells but exerts negligible effects on the growth of Caov-3 cells (13). However, overexpression of IGHG1 did not affect the proliferation or apoptosis of OC cells in this study. This contradiction is probably attributable to differences among the cellular backgrounds or experimental settings. The addition of IGHG1 into the cultured Caov-3 cells failed to alter proliferation probably because the increased IGHG1 level could not activate the relevant signaling pathways. Moreover, the result of IGHG1 treatment in HOC1A cells alone cannot represent other OC cells. Collectively, these results suggest the complex effects of IGHG1 on the proliferation of OC cells. More evidence is required to address this question, such as in vivo growth assays in immunocompromised mice.
Based on the strong correlation between IGHG1 overexpression and OC recurrence (13), we tested whether or not IGHG1 promoted the ability of OC cells to undergo invasion and metastasis, these processes are responsible for almost 90% of cancer-induced deaths (18). We found that IGHG1 overexpression promotes the migration and invasion of OC cells in vitro and positively correlates with the lymph node metastasis in vivo. We showed that IGHG1 overexpression enhances OC cells motility through modulating the expressions of key EMT markers. The critical roles of EMT in cancer stemness, invasion-metastasis cascade, and resistance to therapy are well documented in a wide range of cancers including OC (19-21). Moreover, EMT in OC is regulated by a few molecules and signaling pathways (22-25). Although the mechanistic details underlying the IGHG1-induced EMT have not been determined yet, we speculate that IGHG1 also contributes to stemness and chemo-resistance in OC cells. Further investigations are thus needed to obtain more details about how IGHG1 regulates EMT and the invasion-metastasis cascade in OC.
Conclusions
Our results show that IGHG1 facilitates migration and invasion and regulates several critical EMT markers in OC cells. Impeding the invasion and metastasis of OC cells is a clinical challenge currently; therefore, it is urgent to identify potent diagnostic and therapeutic markers (19). More importantly, COC166-9, which targets IGHG1 specifically, has been showed to be highly effective in the experimental therapies of OC. Targeting IGHG1 is thereby a promising approach to treat metastasis and chemotherapy resistance in OC.
Acknowledgements
This work was supported by Special Funds of the National Natural Science Foundation of China (No. 81341077).
Footnote
Conflicts of Interest: The authors have no conflicts of interest to declare.
References
- Torre LA, Bray F, Siegel RL, et al. Global cancer statistics, 2012. CA Cancer J Clin 2015;65:87–108. [PubMed] DOI:10.3322/caac.21262
- Hogg R, Friedlander M. Biology of epithelial ovarian cancer: implications for screening women at high genetic risk. J Clin Oncol 2004;22:1315–27. [PubMed] DOI:10.1200/JCO.2004.07.179
- Chen W, Zheng R, Zuo T, et al. National cancer incidence and mortality in China, 2012. Chin J Cancer Res 2016;28:1–11. [PubMed] DOI:10.3978/j.issn.1000-9604.2016.02.08
- Siegel RL, Miller KD, Jemal A. Cancer Statistics, 2017. CA Cancer J Clin 2017;67:7–30. [PubMed] DOI:10.3322/caac.21387
- Chen W, Zheng R, Zeng H, et al. Annual report on status of cancer in China, 2011. Chin J Cancer Res 2015;27:2–12. [PubMed] DOI:10.3978/j.issn.1000-9604.2015.01.06
- Jelovac D, Armstrong DK. Recent progress in the diagnosis and treatment of ovarian cancer. CA Cancer J Clin 2011;61:183–203. [PubMed] DOI:10.3322/caac.20113
- Miller DS, Blessing JA, Krasner CN, et al. Phase II evaluation of pemetrexed in the treatment of recurrent or persistent platinum-resistant ovarian or primary peritoneal carcinoma: a study of the Gynecologic Oncology Group. J Clin Oncol 2009;27:2686–91. [PubMed] DOI:10.1200/JCO.2008.19.2963
- Kleppe M, Wang T, Van Gorp T, et al. Lymph node metastasis in stages I and II ovarian cancer: a review. Gynecol Oncol 2011;123:610–4. [PubMed] DOI:10.1016/j.ygyno.2011.09.013
- Nasioudis D, Kanninen TT, Holcomb K, et al. Prevalence of lymph node metastasis and prognostic significance of lymphadenectomy in apparent early-stage malignant ovarian sex cord-stromal tumors. Gynecol Oncol 2017;145:243–7. [PubMed] DOI:10.1016/j.ygyno.2017.03.005
- Scott AM, Wolchok JD, Old LJ. Antibody therapy of cancer. Nat Rev Cancer 2012;12:278–87. [PubMed] DOI:10.1038/nrc3236
- Qian HN. Immunohistological analysis of monoclonal antibody COC166-9 against primary ovarian epithelial cancer. Zhonghua Bing Li Xue Za Zhi (in Chinese) 1988;17:207–9. [PubMed]
- Qian HN, Li WJ. Target therapy of ovarian carcinoma by monoclonal antibodies bearing chemical drugs entrapped in liposomes. Chin Med J (Engl) 1993;106:343–7. [PubMed]
- Ji F, Chang X, Liu C, et al. Prognostic value and characterization of the ovarian cancer-specific antigen CA166-9. Int J Oncol 2015;47:1405–15. [PubMed] DOI:10.3892/ijo.2015.3115
- Liu Z, Tong Y, Liu Y, et al. Effects of suberoylanilide hydroxamic acid (SAHA) combined with paclitaxel (PTX) on paclitaxel-resistant ovarian cancer cells and insights into the underlying mechanisms. Cancer Cell Int 2014;14:112. [PubMed] DOI:10.1186/s12935-014-0112-x
- Ma G, Jing C, Li L, et al. MicroRNA-92b represses invasion-metastasis cascade of esophageal squamous cell carcinoma. Oncotarget 2016;7:20209–22. [PubMed] DOI:10.18632/oncotarget.7747
- Zhang L, Guo L, Tao M, et al. Parasympathetic neurogenesis is strongly associated with tumor budding and correlates with an adverse prognosis in pancreatic ductal adenocarcinoma. Chin J Cancer Res 2016;28:180–6. [PubMed] DOI:10.21147/j.issn.1000-9604.2016.02.05
- Vergara D, Merlot B, Lucot JP, et al. Epithelial-mesenchymal transition in ovarian cancer. Cancer Lett 2010;291:59–66. [PubMed] DOI:10.1016/j.canlet.2009.09.017
- Sethi N, Kang Y. Unravelling the complexity of metastasis — molecular understanding and targeted therapies. Nat Rev Cancer 2011;11:735–48. [PubMed] DOI:10.1038/nrc3125
- Takai M, Terai Y, Kawaguchi H, et al. The EMT (epithelial-mesenchymal-transition)-related protein expression indicates the metastatic status and prognosis in patients with ovarian cancer. J Ovarian Res 2014;7:76. [PubMed] DOI:10.1186/1757-2215-7-76
- Miow QH, Tan TZ, Ye J, et al. Epithelial-mesenchymal status renders differential responses to cisplatin in ovarian cancer. Oncogene 2015;34:1899–907. [PubMed] DOI:10.1038/onc.2014.136
- Gardi NL, Deshpande TU, Kamble SC, et al. Discrete molecular classes of ovarian cancer suggestive of unique mechanisms of transformation and metastases. Clin Cancer Res 2014;20:87–99. [PubMed] DOI:10.1158/1078-0432.CCR-13-2063
- Long H, Xiang T, Qi W, et al. CD133+ ovarian cancer stem-like cells promote non-stem cancer cell metastasis via CCL5 induced epithelial-mesenchymal transition. Oncotarget 2015;6:5846–59. [PubMed] DOI:10.18632/oncotarget.3462
- Wang L, Mezencev R, Švajdler M, et al. Ectopic over-expression of miR-429 induces mesenchymal-to-epithelial transition (MET) and increased drug sensitivity in metastasizing ovarian cancer cells. Gynecol Oncol 2014;134:96–103. [PubMed] DOI:10.1016/j.ygyno.2014.04.055
- Chiu WT, Huang YF, Tsai HY, et al. FOXM1 confers to epithelial-mesenchymal transition, stemness and chemoresistance in epithelial ovarian carcinoma cells. Oncotarget 2015;6:2349–65. [PubMed] DOI:10.18632/oncotarget.2957
- Fidler IJ, Kripke ML. The challenge of targeting metastasis. Cancer Metastasis Rev 2015;34:635–41. [PubMed] DOI:10.1007/s10555-015-9586-9