Immunotherapy for pancreatic ductal adenocarcinoma: an overview of clinical trials
Evolution of tumor immunology
The role of the immune system in the development of neoplastic diseases has been the subject of investigation and controversy for several decades. In 1891, William Coley offered one of the first examples of the efficacy of the immune system in treating cancerous lesions. His strategy consisted of intratumoral injections of live or inactivated Streptococcus pyogenes and Serratia marcenses, known as “Coley’s toxin”. The injected bacteria were capable of initiating a local inflammatory response resulting in activation of antibacterial phagocytes and potential killing of nearby tumor cells by virtue of profound inflammatory response (1). Data derived from Coley’s work were collected for over 40 years and the results of his studies were published in 1953 (2,3). As a result of his pioneering work, Coley is often credited as the father of cancer immunotherapy.
The current view of immune surveillance suggests that cancerous cells are maintained in check by the immune system, which recognizes and eliminates abnormal cells (4-7). The process of immune-surveillance depends on a series of events that are necessary to mount an effective antitumor response (1). Cancer cells express specific epitopes (i.e., neo-antigens) on their cell surface as a result of cancerous transformation (8,9). These epitopes are also known as tumor-associated antigens (TAAs) and are usually captured, processed and presented by dendritic cells (DCs) (10,11). DCs, which are often recognized as the most potent antigen-presenting cells in the human body, require activation and/or maturation signals to differentiate and eventually migrate to regional lymph nodes (12,13). Once in the lymph nodes, mature DCs present TAAs to naive T cells that then undergo expansion and differentiation to become activated T cells. activated T cells eventually leave the lymph nodes and infiltrate into the tumor site where they execute their cytotoxic activity to kill tumor cells (1).
Tumor cells, however, can evade immune control through several complex mechanisms, utilizing immunosuppressive and tolerogenic strategies including immunoediting (14,15). Immunoediting is composed primarily of three sequential stages known as elimination, equilibrium, and escape (7,14,16). During the first phase of “elimination”, cancerous cells are identified and appropriately destroyed by the immune system. During the second phase of “equilibrium,” the immune system prevents further tumor outgrowth but it fails to eliminate cancerous cells completely. The third phase, “escape,” is a direct consequence of the previous two phases, and can be seen as the product of selective pressure of the immune system on cancer cells. In this final phase, cancer cells, which evolve from the original cancerous cell, are now capable of evading the immune surveillance and continue to proliferate.
The pancreatic cancer microenvironment
Pancreatic ductal adenocarcinoma (PDAC) presents several challenges that set it apart from those more immunogenic tumors, such as melanoma and renal cell cancer (17,18). A dysregulation of the immune system is one of the facilitating factors for PDAC development, thus legitimizing the role of the immune network in PDAC (19-22).
One of the principal characteristics of PDAC is the abundance of stromal desmoplasia that constitutes the tumor microenvironment in which the components of the immune network are distributed (23,24). This extensive stromal desmoplasia, also known as fibrosis, has been shown to promote tumor development and most importantly to prevent the penetration and uptake of chemotherapeutic agents (25,26). One of the major players in PDAC desmoplasia is the pancreatic stellate cell (PSC). Stimulated by transforming growth factor β (TGF-β) and platelet-derived growth factor (PDGF), the PSCs initiate a process of synthesis and deposition of extracellular matrix (ECMs) proteins that eventually leads to the extensive desmoplastic reaction seen in PDAC (27,28). Preclinical models have shown that targeting the signaling cascade leading to ECMs protein synthesis could enhance drug penetration in the pancreatic neoplastic tissue (29). However, PDAC clinical trials have yet to show a significant benefit from this approach. In addition, activation of inhibitory T-cell checkpoints (i.e., CTLA-4, PD-1) may have a contributing role as does the particularly hostile tumor microenvironment characterized by abundant stroma that prevents the effector T-cell from functioning in various manners (30).
Several cytokines appear to be dysregulated and contribute to cancer progression in PDAC. In particular, higher levels of circulating interleukin-6 (IL-6) are identified in patients with PDAC and appear to promote cancer progression through enhancement of protumorigenic Stat3 signaling (20,31). Furthermore, members of the IL-1 family [e.g., IL-α, IL-β and IL-1 receptor antagonist (IL-1ra)] seem to play a role in PDAC development (32-34). Immunosuppressive cytokine IL-10 is up regulated in PDAC, which leads to a reduction in effector cell function in the PDAC microenvironment and indicates a worse prognosis (35,36).
Tumor-infiltrating lymphocytes (TILs) have a paramount role in tumor specific cellular adaptive immunity. The main components of this population are CD8+ cytotoxic T cells, CD4+ helper T cells (e.g., Th1, Th2, and Th17), and regulatory T-cells (Tregs) (18). CD8+ T-lymphocytes are the dominant subset of T-lymphocytes in the PDAC microenvironment and their presence is associated with prolonged survival (37-39). CD8+ cytotoxic T-cells recognize TAA peptides associated with major histocompatibility complex class I on tumor cells, resulting in cancer cell destruction. In addition to their direct cytotoxic effect on tumor cells, CD8+ T cells are capable of mobilizing and triggering macrophage tumoricidal activity (18,40,41). The presence of Th1 and Th2 lymphocytes in the tumor microenvironment appears to have opposite prognostic significance in the setting of PDAC progression (42,43). In fact, the presence of Th1 is associated with favorable prognosis while a predominant infiltration of Th2 and its related cytokines (IL-4, IL-5 or IL-13) often correlates with disease progression (18). Of interest is the role of IL-5 and IL-13, these cytokines likely stimulate the desmoplastic reaction increasing ECM deposition and collagen synthesis (44). Furthermore, IL-13 appears to downregulate proinflammatory cytokines (IL-1, IL-6, TNF-α) and chemokines, and effectively inhibits antibody-dependent cellular toxicity (45,46). Nevertheless, IL-13 acts as an autocrine growth factor for PDAC (47,48). Regulatory T-cells (Tregs), which are positive for CD4+, CD25+, and Foxp3, are enriched in the tumor microenvironment (49,50). Tregs effectively suppress the adaptive immune response and their presence in the tumor microenvironment leads to a decreased presence of CD8+ T-cells and often correlates with poor prognosis (50,51). Other cell types, like myeloid-derived suppressive cells (MDSCs) and neutrophils, also participate in the immune reaction during the development and progression of PDAC resulting in dynamic interactions between the tumor cells, and the immune system.
Strategies of cancer immunotherapy
Different strategies for cancer immunotherapy have been proposed and investigated. These therapeutic strategies can be grouped into active or passive, based on the involvement of the host immune system. Active immunotherapy aims to stimulate the host immune response to recognize TAAs and eventually destroy tumor cells. This often requires administration of cytokines, immunomodulatory agents, or therapeutic vaccines that eventually lead to the expansion of tumor-specific T cells. Passive immunotherapy requires the exogenous administration of activated lymphocytes (e.g., tumor-specific immune effector cells) or antibodies that mediate an immune response (52).
Overview of clinical trials in PDAC immunotherapy
Results from recent clinical trials conducted between 2005 and 2015 are summarized in Table 1. In addition, trials conducted between 2010 and 2015 are discussed in the following sections.
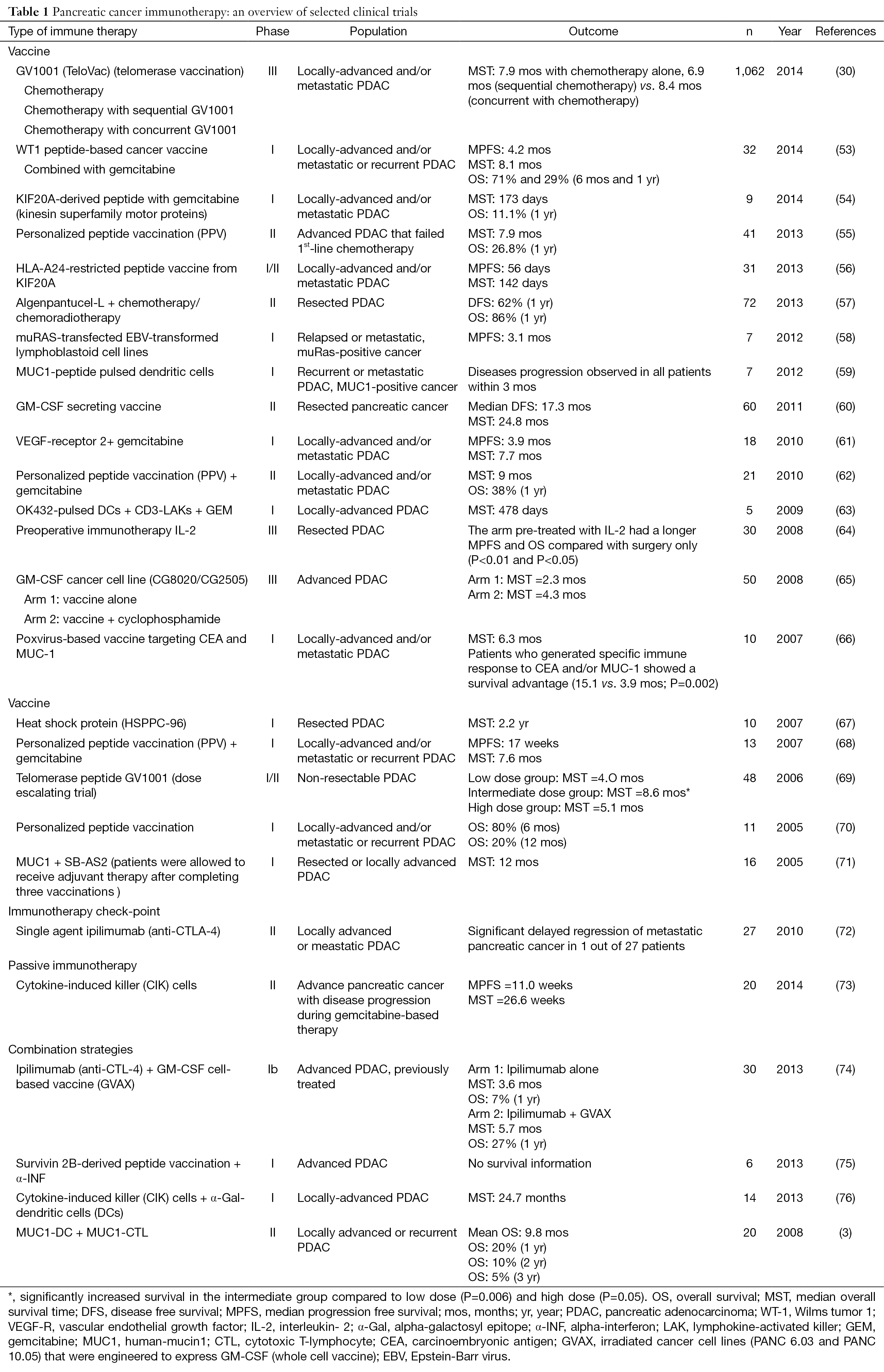
Full table
Adoptive therapy
In one of the most recent phase II trials, Chung et al. evaluated the use of adoptive immunotherapy in patients with advanced pancreatic cancer who experienced disease progression during gemcitabine-based chemotherapy (73). In this study, the authors utilized ex vivo expanded, cytokine-induced killer (CIK) cells (i.e., heterogenous cell population containing >20% of CD3+ CD56+ cells) previously shown to have cytolytic activity in a major histocompatibility complex (MHC)-unrestricted manner (77). Patients enrolled in this study received CIK as the sole cancer therapy. The authors reported a median estimated progression free survival (PFS) of 11.0 weeks and a median estimated overall survival (OS) of 26.6 weeks, which were similar to prior studies using conventional cytotoxic chemotherapy (73,78-80).
Cancer vaccines
Cancer vaccines aim to stimulate the immune system to produce tumor-specific T cells and B cells (81). The primary mechanism of action of therapeutic cancer vaccines is their capacity to increase the presentation of TAAs to the immune system. Generally vaccines can be classified in three major approaches: cell-based vaccines, protein/peptide vaccines, and genetic vaccines. Each strategy has been well-investigated, and each seems to have its own advantages and disadvantages (Figure 1).
Table 2 summarizes the most common cellular targets utilized in recent clinical trials of PDAC cancer vaccines, including: telomerase, Wilms tumor gene, KIF20A, alpha-galactosyl (α-Gal), survivin, mutated Ras protein, human mucin MUC1 protein, and vascular endothelial growth factor receptor 2 (VEGFR2).
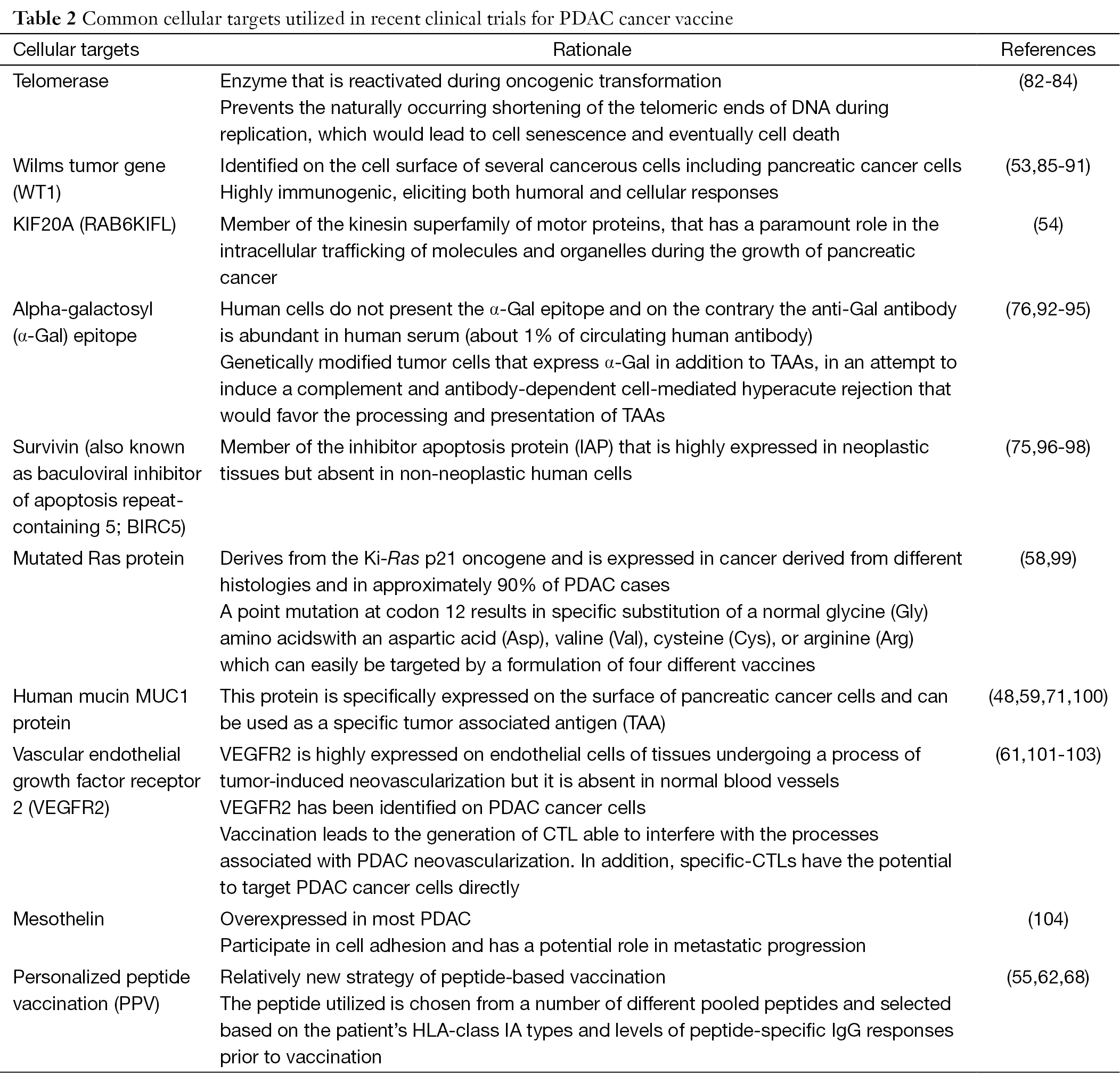
Full table
The TeloVac study is one of the largest randomized, phase III clinical trials to evaluate the efficacy of cancer vaccine in PDAC (30). This trial was conducted in 51 hospitals in the United Kingdom and enrolled 1,062 subjects. It aimed to assess the efficacy and safety of sequential or simultaneous telomerase vaccination (GV1001) in combination with chemotherapy in patients with locally advanced or metastatic pancreatic cancer. Results showed that adding GV1001 vaccine either simultaneously or sequentially to a standard treatment regimen of gemcitabine and capecitabine did not improve OS. The authors suggest that the lack of response seen in this trial may be due to the characteristic rapid progression of pancreatic cancer to metastatic disease, which could prevent an active immune response from developing.
Active peptide-based immunotherapy utilizing Wilms tumor (WT1) protein has been investigated in combination with gemcitabine for patients with advanced pancreatic cancer (53). In this phase I clinical trial, vaccination with WT1 in combination with gemcitabine was found to be safe. Furthermore, although the trial was not designed to evaluate survival benefit, it appears that the patients in whom a WT1 specific immunity was induced had better clinical outcomes translating to a 12-month or longer survival time and an improved quality of life (QOL).
Suzuki et al. conducted the first phase I trial aimed to investigate the use of a vaccine composed of an epitope peptide KIF20A in combination with gemcitabine in patients with advanced pancreatic cancer (unresectable and/or metastatic) who had already received prior conventional chemotherapy and/or radiotherapy (54). The authors reported no adverse events directly attributable to the vaccine and demonstrated enhancement of INF-γ-producing cells in 8 out of the 9 patients enrolled (54).
The enthusiasm that followed two trials conducted by Yanagimoto et al., aimed at the evaluation of personalized peptide vaccination (PPV) in combination with gemcitabine (62,68), prompted Yutani et al. to test this vaccination strategy in a phase II trial in patients with chemotherapy–resistant advanced pancreatic cancer (55). Patients enrolled in this trial had a median survival time (MST) of 7.9 months with a 1-year survival rate of 26.8%. However the authors noted that patients who were treated solely with PPV (n=8) had a MST of 3.1 months compared to patients who received PPV vaccination combined with chemotherapy (9.6 months; P=0.0013). Therefore, Yutani et al. concluded that PPV offers no advantages as a single therapy in patients with advanced PDAC, although its use combined with chemotherapy could positively influence OS.
Algenpantucel-L (NewLink Genetics Corporation, Ames, IA, USA) is an allogenic cancer vaccine composed of two human PDAC cell lines (HAPa-1 and HAPa-2) (57). These cells express the α[1,3]-galactosyl epitopes (α-Gal) as a result of genetic engineering processes. Injection of algenpantucel-L generates a hyperacute rejection that ultimately stimulates the patient’s immune system to target the existing PDAC lesions (57,105). In the phase II trial conducted by Hardacre et al., algenpantucel-L was administered in combination with standard chemotherapy and chemoradiotherapy (gemcitabine + 5-fluorouracile-based chemoradiotherapy) as adjuvant treatment following surgical resection of a primary PDAC lesion. Results from this trial were encouraging; with a reported 12-month disease free-survival of 62% and 12-month OS of 86% with a median follow-up of 21 months. The authors remarked that the percentage of patients surviving at 12-month was higher than survival predicted by the widely accepted prognostic nomogram described by Brennan et al. (86% vs. 55-63%) (57). Another positive note was that patients treated with algenpantucel-L experienced minimal side effects, mainly consisting of injection site pain and induration. Although several interesting findings emerged from this study, its results should be interpreted carefully as no definitive conclusion was achieved on the advantage provided by the addition of algenpantucel-L to standard chemotherapy regimens.
Asahara et al. conducted a non-randomized, open-label, phase I/II clinical trial utilizing the KIF20A-66 epitope restricted to the HLA-A2402 (the most common HLA-A allele in the Japanese population enrolled in the study). The KIF20A-66 is a member of the kinase superfamily protein (see above) that is highly expressed in pancreatic cancer cells. Patients with advanced PDAC who failed gemcitabine-based therapy comprised the cohort selected for this trial. Median survival time was compared to a historic cohort and patients treated with cancer vaccine therapy showed an overall median survival time of 142 days compared to 83 days (P=0.0468) of the historic cohort. Interestingly, the authors reported the case of one patient who experienced complete response with resolution of liver metastatic lesion. This patient was noted to have a strong cytotoxic T-cell (CTL) response to KIF20A-66 epitope that remained detectable even 2 years from the last dose of vaccine administration (56).
Kubuschol et al. investigated the use of an autologous lymphoblastoid cell line (LCL)-based vaccine. LCLs are “professional” antigen presenting cells (APCs) characterized by a very high immunostimulatory capacity that are easily obtained from EBV-positive patients. These cells are a particularly attractive source of APCs because they are characterized by a rapid growth in vitro providing an easily accessible cell pool (58). In this trial LCLs where engineered to express a mutated Ras-protein on the cell surface (muRas-LCL). Patients enrolled in the study, received weekly subcutaneous injections with muRac-LCL vaccine. Tumor specific T-cell response (muRas-specific) was observed in six of the seven patients enrolled in the trial (85%). However, despite an initial clinical response observed in 57% of cases, after 4 months from initial vaccination, all patients showed disease progression. One of the most important findings of this study was that the use of tumor antigen-transfected LCL proved to be an efficient alternative to DCs to serve in the role of APCs for future vaccine trials (58).
Rong et al. investigated the immunological response induced by the administration of MUC1-peptide-pulsed DCs-based vaccine in a cohort of advanced PDAC patients (59). Patients were selected based on tumor expression of MUC1. Patients’ autologous DCs were collected, pulsed with MUC1-peptide and injected intradermally for three to four administrations. Although the vaccination regimen was safe, evidence of a significant immune response was observed in only two of the seven patients enrolled.
Lutz et al. conducted a phase II clinical trial enrolling 60 patients with resected pancreatic adenocarcinoma (60). In their trial, the authors utilized an allogenic granulocyte-macrophage colony stimulating factor-secreting tumor vaccine (GM-CSF), based on cancer cell lines PANC 10.05 and PANC 6.03, injected directly into lymph node regions. The initial vaccine dose was followed by 5-FU based chemoradiotherapy and additional vaccine doses were given after chemotherapy completion in patients that remained disease free. Patients that completed all 4 doses of the vaccine therapy received a final vaccine booster 6 months after the administration of the fourth dose. The first observation from the study was that the regimen of vaccination with GM-CSF-secreting tumor cells following adjuvant chemoradiotherapy was well tolerated. In fact, no local or dose-limiting toxicities were observed. Additionally, when the study cohort was compared to a historical cohort treated at the same institution, the authors found no significant difference in the median OS (HR: 0.96, 95% CI, 0.68-1.35, P=0.8).
Miyazawa et al. investigated the use of a peptide vaccine for human vascular endothelial growth factor receptor 2 (VEGFR-2) in combination with gemcitabine adjuvant therapy (61). In this phase I clinical trial, 21 patients with advanced pancreatic cancer were enrolled and 18 patients were able to complete the vaccination schedule and were evaluated in their final analysis. Although the treatment was well tolerated, and specific CTL response against the vaccinated peptide was observed in the majority of the treated patients (61%), no correlation of CTL response and overall clinical outcome was appreciated. Following the results of this study a new double-blind, placebo-controlled trial was designed to investigate the role of an oral VEGFR-2 vaccine in patients with stage IV and locally advanced pancreatic cancer. The study is currently ongoing (NCT01486329) (106).
The use of GVAX, a whole-cell vaccine composed of two irradiated cancer cell lines (PANC 6.03 and PANC 10.05) engineered to express GM-CSF has been investigated in multiple phase I and II studies. Early studies showed that vaccination with GVAX leads to induction of CD8+ T-cell responses against multiple mesothelin-specific epitopes that has been shown to correlate with improved survival (60,65,107).
Although designed to evaluate a mixed cohort with advanced solid tumor, the study conducted by Le and colleagues offered interesting results on the use of Listeria-based vaccines (108). Live-Attenuated Listeria vaccines are used based on the ability of Listeria monocytogenes (Lm) to stimulate both innate and adaptive immunity. After administration, Lm is phagocytized in the liver and generates a local inflammatory response leading to the activation and recruitment of natural killer (NK) and T cells. Le and colleagues, investigated the use of live-attenuated Lm-based vaccines in two cohort of patients with liver metastasis originated from PDAC (108). In the first phase of their study, the safety and efficacy of the use of Lm-based vaccine (ANZ-100) was tested and found to be acceptable. Following these initial findings, Lm was modified to express human mesothelin (CRS-207), a tumor associated antigen (TAA) known to be expressed by PDAC. The ultimate goal was to induce an immune response that would produce tumor antigen-specific T cells directed toward PDAC expressing human mesothelin protein. Three of the seven patients treated with (CRS-207) survived more than 15 months and showed specific T-cell response to the vaccine component listeriolysin O (LLO), although all three patients had received prior immunotherapy with GM-CSF-based whole-cell vaccine (GVAX) which confounds the overall results. Unfortunately, LLO-response was not evaluated in the remaining patients who survived less than 15 months.
Taken together these results suggest that cancer vaccines are in general well tolerated and able to generate an immune response directed toward specific cancer targets. However, with the exception of some isolated but remarkable clinical responses, the impact of cancer vaccines on OS in PDAC appears to be minimal for the majority of patients. Several explanations for this lack of efficacy have been proposed. It is worth noting that advanced stages of PDAC are characterized by rapid disease progression that might not allow enough time for the immune system to mount an effective response that often requires weeks to months to develop.
Immune checkpoint blockade
T cell response can be controlled by a few cosignaling receptors with inhibitory functions, now known as immune checkpoints, which include CTLA-4, PD-1 and BTLA. Agents blocking these molecules are able to unleash endogenous anti-tumor T cell responses, so as to limit tumor growth (109). Royal et al. investigated the role of single agent Ipilimumab, an anti-CTLA-4 antibody, in a cohort of locally advanced or metastatic pancreatic adenocarcinoma (72). Ipilimumab has been previously effective in the treatment of melanoma, renal cell carcinoma, and prostate cancer (110-112). CTLA-4 is transiently expressed on the T-cell surface following activation and leads to a decrease in T-cell response following its binding to B7-1 or B7-2 on APCs or target tissue (113). In this phase 2 trial, the authors observed a significant delayed regression of metastatic pancreatic cancer in one out of the twenty-seven patients enrolled in the study. The findings of this phase 2 trial were particularly interesting as they underlined the mechanism of action of Ipilimumab represented by immunomodulation rather than direct tumoricidal activity. In fact, the patient who showed a response to Ipilimumab treatment had initially experienced marked progression of the disease. The authors concluded that Ipilimumab alone might not be a valuable treatment for advanced pancreatic cancer, however they laid the basis for future trials of combination therapy with immune checkpoint blockade combined with vaccine or chemotherapy (72).
Combination immunotherapy trials
Cancer vaccine and immune checkpoint blockade
Although the study conducted by Royal et al. (phase II trial) showed minimal efficacy of anti-CTLA-4 (Ipilimumab) therapy on advanced pancreatic cancer, one patient enrolled in this initial trial showed a significant delayed response suggesting a possible role for immune checkpoint blockade in PDAC (72). Several preclinical studies suggest a possible synergistic role of cancer vaccine therapy that stimulates the immune system and the use of immune checkpoint blockade to allow for the unopposed effector function of cytotoxic T-cells (114,115). On this premise, Le et al. conducted a phase Ib, open-label, randomized study to the determine the safety profile of ipilimumab alone or in combination with GVAX in patients with previously treated PDAC (74). This study showed that the use of Ipulimumab in PDAC patients, with or without GM-CSF-based cell therapy, has an acceptable side effect profile. Induction of immune response was observed as a result of the treatment regimen and correlated with clinical activity, although prolonged treatment appears to be required to obtain a clinical response in the setting of advanced PDAC disease (74). One of the most interesting aspects of this study was the difference in 12-month OS of 27% vs. 7% and the median OS of 5.7 vs. 3.6 months (HR =0.51; P=0.072) respectively for combination therapy vs. monotherapy. Although the trial was not designed to show significant survival differences, the results obtained point to a superiority of the combination therapy over monotherapy (74).
Active immune therapy combined with passive immune therapy
Qiu et al. investigated the use of a combination of DC-based and CIK-based therapy (76). In this study, DCs were initially pulsed with patients’ primary pancreatic carcinoma cells previously transfected in vitro to express α-Gal epitope and opsonized with anti-Gal IgG. This approach enhances the antigenicity of TAAs and facilitates phagocytosis by DCs (76). Subsequently, DCs were co-cultured with CIKs derived from bone marrow stem cells, ultimately generating tumor specific immune responders cells ex vivo (76). The generated CIKs and the mature DCs were then injected in 14 patients with inoperable stage III/IV pancreatic adenocarcinoma. The authors reported a significant increase in patients’ cellular immunity, especially in the percentage of cytotoxic T cells (CD3+CD8+), activated and memory T cells (CD3+CD45RO+), and activated T and NK cells (CD3+CD56+). Furthermore, no serious side effects were experienced during treatment and the reported median OS was 24.7 months (108.1±35.1 weeks), higher than the usual survival reported in the literature for unresectable stage III/IV PDAC.
Kameshima et al. investigated the use of a vaccination protocol of survivin-2B80-88 plus incomplete Freud’s adjuvant (IFA) and α-interferon (INFα) based on favorable results previously obtained in the treatment of colon cancer (75,116,117). The authors reported that more than 50% of the treated patients showed positive clinical and immunological response.
Immunotherapy combined with chemotherapy
Algenpantucel-L is currently being investigated in an open label, phase III, randomized trial in combination with FOLFIRINOX (oxaliplatin, 5-FU, irinotecan, and leucovorin) in patients with borderline resectable or locally advanced pancreatic cancer (NCT01836432). The estimated primary completion date is September 2015. This is currently the first study that is using a FOLFIRINOX based chemotherapy.
Conclusions and prospective
Traditional treatments for PDAC are limited and ineffective, and novel therapeutic strategies are greatly needed. Despite recent advancements in systemic chemotherapeutic regimens, the median survival time of advanced pancreatic cancer patients remains 4-11 months (118-121). The identification and development of more efficacious therapies is of paramount importance. Immunotherapy offers encouraging results in preclinical models but often fails to show clear benefits in clinical trials for PDAC. Immunotherapy, as a single treatment strategy, might not be sufficient to effectively treat PDAC. For example, evidence suggests that active immunotherapy should be used in combination with traditional chemotherapy and/or radiotherapy or even in combination with other forms of immune therapy (e.g., immune checkpoint blockade or passive immune therapy) (122). This strategy could take advantage of the various effects traditional chemotherapeutic agents and/or radiotherapy exert on the immune system (123,124). Acting through direct killing of cancerous cells, chemotherapeutic agents indirectly lead to the release of pro-inflammatory molecules and TAAs (85). In addition, chemotherapy can suppress the inhibitory mechanism in the tumor microenvironment. In fact, reduction of the number of Tregs cells and myeloid derived suppressor cells (MDSC) and their related cytokines (IL-17 and IL-15) are one of the recognized positive effects of chemotherapy on tumor microenvironment. This change in the composition of cells in the tumor microenvironment could facilitate the development of a more efficacious effector immune response against cancer cells (52,122,125). However, the potential synergistic effects of chemotherapy have to be balanced with its potential immunosuppressive effects. Future studies should focus on identifying appropriate dosing and timing of synergistic chemotherapy administration in order to mitigate its immunosuppressive effects and maximize the effect of immunotherapeutic cancer treatments. Several aspects remain to be clarified in PDAC cancer immunotherapy, including optimal cellular targets, delivery vectors for cancer vaccines, combination with existing treatment strategies, and patient selection. Future clinical trials should be designed to address these unresolved aspects of PDAC immunotherapy.
Acknowledgements
Funding: This work is partially supported by American Cancer Society IRG 57-001-53.
Footnote
Conflicts of Interest: The authors have no conflicts of interest to declare.
References
- Mellman I, Coukos G, Dranoff G. Cancer immunotherapy comes of age. Nature 2011;480:480-9. [PubMed]
- Nauts HC, Fowler GA, Bogatko FH. A review of the influence of bacterial infection and of bacterial products (Coley's toxins) on malignant tumors in man; a critical analysis of 30 inoperable cases treated by Coley's mixed toxins, in which diagnosis was confirmed by microscopic examination selected for special study. Acta Med Scand Suppl 1953;276:1-103. [PubMed]
- Kondo H, Hazama S, Kawaoka T, et al. Adoptive immunotherapy for pancreatic cancer using MUC1 peptide-pulsed dendritic cells and activated T lymphocytes. Anticancer Res 2008;28:379-87. [PubMed]
- Hanahan D, Weinberg RA. Hallmarks of cancer: the next generation. Cell 2011;144:646-74. [PubMed]
- Finn OJ. Immuno-oncology: understanding the function and dysfunction of the immune system in cancer. Ann Oncol 2012;23:viii6-9.
- Disis ML. Immune regulation of cancer. J Clin Oncol 2010;28:4531-8. [PubMed]
- Disis ML. Mechanism of action of immunotherapy. Semin Oncol 2014;41:S3-13. [PubMed]
- Segal NH, Parsons DW, Peggs KS, et al. Epitope landscape in breast and colorectal cancer. Cancer Res 2008;68:889-92. [PubMed]
- Boon T, Coulie PG, Van den Eynde BJ, et al. Human T cell responses against melanoma. Annu Rev Immunol 2006;24:175-208. [PubMed]
- Trombetta ES, Mellman I. Cell biology of antigen processing in vitro and in vivo. Annu Rev Immunol 2005;23:975-1028. [PubMed]
- Mellman I, Steinman RM. Dendritic cells: specialized and regulated antigen processing machines. Cell 2001;106:255-8. [PubMed]
- Randolph GJ. Dendritic cell migration to lymph nodes: cytokines, chemokines, and lipid mediators. Semin Immunol 2001;13:267-74. [PubMed]
- Itano AA, McSorley SJ, Reinhardt RL, et al. Distinct dendritic cell populations sequentially present antigen to CD4 T cells and stimulate different aspects of cell-mediated immunity. Immunity 2003;19:47-57. [PubMed]
- Dunn GP, Koebel CM, Schreiber RD. Interferons, immunity and cancer immunoediting. Nat Rev Immunol 2006;6:836-48. [PubMed]
- Janikashvili N, Bonnotte B, Katsanis E, et al. The dendritic cell-regulatory T lymphocyte crosstalk contributes to tumor-induced tolerance. Clin Dev Immunol 2011;2011:430394.
- Vesely MD, Schreiber RD. Cancer immunoediting: antigens, mechanisms, and implications to cancer immunotherapy. Ann N Y Acad Sci 2013;1284:1-5. [PubMed]
- Inman KS, Francis AA, Murray NR. Complex role for the immune system in initiation and progression of pancreatic cancer. World J Gastroenterol 2014;20:11160-81. [PubMed]
- Wörmann SM, Diakopoulos KN, Lesina M, et al. The immune network in pancreatic cancer development and progression. Oncogene 2014;33:2956-67. [PubMed]
- Moses AG, Maingay J, Sangster K, et al. Pro-inflammatory cytokine release by peripheral blood mononuclear cells from patients with advanced pancreatic cancer: relationship to acute phase response and survival. Oncol Rep 2009;21:1091-5. [PubMed]
- Lesina M, Kurkowski MU, Ludes K, et al. Stat3/Socs3 activation by IL-6 transsignaling promotes progression of pancreatic intraepithelial neoplasia and development of pancreatic cancer. Cancer Cell 2011;19:456-69. [PubMed]
- Sakamoto H, Kimura H, Sekijima M, et al. Plasma concentrations of angiogenesis-related molecules in patients with pancreatic cancer. Jpn J Clin Oncol 2012;42:105-12. [PubMed]
- Hill KS, Gaziova I, Harrigal L, et al. Met receptor tyrosine kinase signaling induces secretion of the angiogenic chemokine interleukin-8/CXCL8 in pancreatic cancer. PLoS One 2012;7:e40420. [PubMed]
- Tjomsland V, Niklasson L, Sandström P, et al. The desmoplastic stroma plays an essential role in the accumulation and modulation of infiltrated immune cells in pancreatic adenocarcinoma. Clin Dev Immunol 2011;2011:212810.
- Zischek C, Niess H, Ischenko I, et al. Targeting tumor stroma using engineered mesenchymal stem cells reduces the growth of pancreatic carcinoma. Ann Surg 2009;250:747-53. [PubMed]
- Minchinton AI, Tannock IF. Drug penetration in solid tumours. Nat Rev Cancer 2006;6:583-92. [PubMed]
- Netti PA, Berk DA, Swartz MA, et al. Role of extracellular matrix assembly in interstitial transport in solid tumors. Cancer Res 2000;60:2497-503. [PubMed]
- Yen TW, Aardal NP, Bronner MP, et al. Myofibroblasts are responsible for the desmoplastic reaction surrounding human pancreatic carcinomas. Surgery 2002;131:129-34. [PubMed]
- Akhurst RJ, Hata A. Targeting the TGFβ signalling pathway in disease. Nat Rev Drug Discov 2012;11:790-811. [PubMed]
- Olive KP, Jacobetz MA, Davidson CJ, et al. Inhibition of Hedgehog signaling enhances delivery of chemotherapy in a mouse model of pancreatic cancer. Science 2009;324:1457-61. [PubMed]
- Middleton G, Silcocks P, Cox T, et al. Gemcitabine and capecitabine with or without telomerase peptide vaccine GV1001 in patients with locally advanced or metastatic pancreatic cancer (TeloVac): an open-label, randomised, phase 3 trial. Lancet Oncol 2014;15:829-40. [PubMed]
- Okada S, Okusaka T, Ishii H, et al. Elevated serum interleukin-6 levels in patients with pancreatic cancer. Jpn J Clin Oncol 1998;28:12-5. [PubMed]
- Tjomsland V, Spångeus A, Välilä J, et al. Interleukin 1α sustains the expression of inflammatory factors in human pancreatic cancer microenvironment by targeting cancer-associated fibroblasts. Neoplasia 2011;13:664-75. [PubMed]
- Ling J, Kang Y, Zhao R, et al. KrasG12D-induced IKK2/β/NF-κB activation by IL-1α and p62 feedforward loops is required for development of pancreatic ductal adenocarcinoma. Cancer Cell 2012;21:105-20. [PubMed]
- Müerköster S, Wegehenkel K, Arlt A, et al. Tumor stroma interactions induce chemoresistance in pancreatic ductal carcinoma cells involving increased secretion and paracrine effects of nitric oxide and interleukin-1beta. Cancer Res 2004;64:1331-7. [PubMed]
- Bellone G, Smirne C, Mauri FA, et al. Cytokine expression profile in human pancreatic carcinoma cells and in surgical specimens: implications for survival. Cancer Immunol Immunother 2006;55:684-98. [PubMed]
- Poch B, Lotspeich E, Ramadani M, et al. Systemic immune dysfunction in pancreatic cancer patients. Langenbecks Arch Surg 2007;392:353-8. [PubMed]
- Ademmer K, Ebert M, Müller-Ostermeyer F, et al. Effector T lymphocyte subsets in human pancreatic cancer: detection of CD8+CD18+ cells and CD8+CD103+ cells by multi-epitope imaging. Clin Exp Immunol 1998;112:21-6. [PubMed]
- Ino Y, Yamazaki-Itoh R, Shimada K, et al. Immune cell infiltration as an indicator of the immune microenvironment of pancreatic cancer. Br J Cancer 2013;108:914-23. [PubMed]
- Fukunaga A, Miyamoto M, Cho Y, et al. CD8+ tumor-infiltrating lymphocytes together with CD4+ tumor-infiltrating lymphocytes and dendritic cells improve the prognosis of patients with pancreatic adenocarcinoma. Pancreas 2004;28:e26-31. [PubMed]
- Smyth MJ, Dunn GP, Schreiber RD. Cancer immunosurveillance and immunoediting: the roles of immunity in suppressing tumor development and shaping tumor immunogenicity. Adv Immunol 2006;90:1-50. [PubMed]
- Schreiber RD, Pace JL, Russell SW, et al. Macrophage-activating factor produced by a T cell hybridoma: physiochemical and biosynthetic resemblance to gamma-interferon. J Immunol 1983;131:826-32. [PubMed]
- Fridman WH, Pagès F, Sautès-Fridman C, et al. The immune contexture in human tumours: impact on clinical outcome. Nat Rev Cancer 2012;12:298-306. [PubMed]
- De Monte L, Reni M, Tassi E, et al. Intratumor T helper type 2 cell infiltrate correlates with cancer-associated fibroblast thymic stromal lymphopoietin production and reduced survival in pancreatic cancer. J Exp Med 2011;208:469-78. [PubMed]
- Wynn TA. Fibrotic disease and the T(H)1/T(H)2 paradigm. Nat Rev Immunol 2004;4:583-94. [PubMed]
- Skinnider BF, Elia AJ, Gascoyne RD, et al. Interleukin 13 and interleukin 13 receptor are frequently expressed by Hodgkin and Reed-Sternberg cells of Hodgkin lymphoma. Blood 2001;97:250-5. [PubMed]
- Zurawski G, de Vries JE. Interleukin 13, an interleukin 4-like cytokine that acts on monocytes and B cells, but not on T cells. Immunol Today 1994;15:19-26. [PubMed]
- Formentini A, Prokopchuk O, Sträter J, et al. Interleukin-13 exerts autocrine growth-promoting effects on human pancreatic cancer, and its expression correlates with a propensity for lymph node metastases. Int J Colorectal Dis 2009;24:57-67. [PubMed]
- Kornmann M, Kleeff J, Debinski W, et al. Pancreatic cancer cells express interleukin-13 and -4 receptors, and their growth is inhibited by Pseudomonas exotoxin coupled to interleukin-13 and -4. Anticancer Res 1999;19:125-31. [PubMed]
- Curiel TJ, Coukos G, Zou L, et al. Specific recruitment of regulatory T cells in ovarian carcinoma fosters immune privilege and predicts reduced survival. Nat Med 2004;10:942-9. [PubMed]
- Hiraoka N, Onozato K, Kosuge T, et al. Prevalence of FOXP3+ regulatory T cells increases during the progression of pancreatic ductal adenocarcinoma and its premalignant lesions. Clin Cancer Res 2006;12:5423-34. [PubMed]
- Clark EJ, Connor S, Taylor MA, et al. Preoperative lymphocyte count as a prognostic factor in resected pancreatic ductal adenocarcinoma. HPB (Oxford) 2007;9:456-60. [PubMed]
- Melero I, Gaudernack G, Gerritsen W, et al. Therapeutic vaccines for cancer: an overview of clinical trials. Nat Rev Clin Oncol 2014;11:509-24. [PubMed]
- Nishida S, Koido S, Takeda Y, et al. Wilms tumor gene (WT1) peptide-based cancer vaccine combined with gemcitabine for patients with advanced pancreatic cancer. J Immunother 2014;37:105-14. [PubMed]
- Suzuki N, Hazama S, Ueno T, et al. A phase I clinical trial of vaccination with KIF20A-derived peptide in combination with gemcitabine for patients with advanced pancreatic cancer. J Immunother 2014;37:36-42. [PubMed]
- Yutani S, Komatsu N, Yoshitomi M, et al. A phase II study of a personalized peptide vaccination for chemotherapy-resistant advanced pancreatic cancer patients. Oncol Rep 2013;30:1094-100. [PubMed]
- Asahara S, Takeda K, Yamao K, et al. Phase I/II clinical trial using HLA-A24-restricted peptide vaccine derived from KIF20A for patients with advanced pancreatic cancer. J Transl Med 2013;11:291. [PubMed]
- Hardacre JM, Mulcahy M, Small W, et al. Addition of algenpantucel-L immunotherapy to standard adjuvant therapy for pancreatic cancer: a phase 2 study. J Gastrointest Surg 2013;17:94-100;discussion 100-1. [PubMed]
- Kubuschok B, Pfreundschuh M, Breit R, et al. Mutated Ras-transfected, EBV-transformed lymphoblastoid cell lines as a model tumor vaccine for boosting T-cell responses against pancreatic cancer: a pilot trial. Hum Gene Ther 2012;23:1224-36. [PubMed]
- Rong Y, Qin X, Jin D, et al. A phase I pilot trial of MUC1-peptide-pulsed dendritic cells in the treatment of advanced pancreatic cancer. Clin Exp Med 2012;12:173-80. [PubMed]
- Lutz E, Yeo CJ, Lillemoe KD, et al. A lethally irradiated allogeneic granulocyte-macrophage colony stimulating factor-secreting tumor vaccine for pancreatic adenocarcinoma. A Phase II trial of safety, efficacy, and immune activation. Ann Surg 2011;253:328-35. [PubMed]
- Miyazawa M, Ohsawa R, Tsunoda T, et al. Phase I clinical trial using peptide vaccine for human vascular endothelial growth factor receptor 2 in combination with gemcitabine for patients with advanced pancreatic cancer. Cancer Sci 2010;101:433-9. [PubMed]
- Yanagimoto H, Shiomi H, Satoi S, et al. A phase II study of personalized peptide vaccination combined with gemcitabine for non-resectable pancreatic cancer patients. Oncol Rep 2010;24:795-801. [PubMed]
- Hirooka Y, Itoh A, Kawashima H, et al. A combination therapy of gemcitabine with immunotherapy for patients with inoperable locally advanced pancreatic cancer. Pancreas 2009;38:e69-74. [PubMed]
- Caprotti R, Brivio F, Fumagalli L, et al. Free-from-progression period and overall short preoperative immunotherapy with IL-2 increases the survival of pancreatic cancer patients treated with macroscopically radical surgery. Anticancer Res 2008;28:1951-4. [PubMed]
- Laheru D, Lutz E, Burke J, et al. Allogeneic granulocyte macrophage colony-stimulating factor-secreting tumor immunotherapy alone or in sequence with cyclophosphamide for metastatic pancreatic cancer: a pilot study of safety, feasibility, and immune activation. Clin Cancer Res 2008;14:1455-63. [PubMed]
- Kaufman HL, Kim-Schulze S, Manson K, et al. Poxvirus-based vaccine therapy for patients with advanced pancreatic cancer. J Transl Med 2007;5:60. [PubMed]
- Maki RG, Livingston PO, Lewis JJ, et al. A phase I pilot study of autologous heat shock protein vaccine HSPPC-96 in patients with resected pancreatic adenocarcinoma. Dig Dis Sci 2007;52:1964-72. [PubMed]
- Yanagimoto H, Mine T, Yamamoto K, et al. Immunological evaluation of personalized peptide vaccination with gemcitabine for pancreatic cancer. Cancer Sci 2007;98:605-11. [PubMed]
- Bernhardt SL, Gjertsen MK, Trachsel S, et al. Telomerase peptide vaccination of patients with non-resectable pancreatic cancer: A dose escalating phase I/II study. Br J Cancer 2006;95:1474-82. [PubMed]
- Yamamoto K, Mine T, Katagiri K, et al. Immunological evaluation of personalized peptide vaccination for patients with pancreatic cancer. Oncol Rep 2005;13:874-83. [PubMed]
- Ramanathan RK, Lee KM, McKolanis J, et al. Phase I study of a MUC1 vaccine composed of different doses of MUC1 peptide with SB-AS2 adjuvant in resected and locally advanced pancreatic cancer. Cancer Immunol Immunother 2005;54:254-64. [PubMed]
- Royal RE, Levy C, Turner K, et al. Phase 2 trial of single agent Ipilimumab (anti-CTLA-4) for locally advanced or metastatic pancreatic adenocarcinoma. J Immunother 2010;33:828-33. [PubMed]
- Chung MJ, Park JY, Bang S, et al. Phase II clinical trial of ex vivo-expanded cytokine-induced killer cells therapy in advanced pancreatic cancer. Cancer Immunol Immunother 2014;63:939-46. [PubMed]
- Le DT, Lutz E, Uram JN, et al. Evaluation of ipilimumab in combination with allogeneic pancreatic tumor cells transfected with a GM-CSF gene in previously treated pancreatic cancer. J Immunother 2013;36:382-9. [PubMed]
- Kameshima H, Tsuruma T, Kutomi G, et al. Immunotherapeutic benefit of α-interferon (IFNα) in survivin2B-derived peptide vaccination for advanced pancreatic cancer patients. Cancer Sci 2013;104:124-9. [PubMed]
- Qiu Y, Yun MM, Xu MB, et al. Pancreatic carcinoma-specific immunotherapy using synthesised alpha-galactosyl epitope-activated immune responders: findings from a pilot study. Int J Clin Oncol 2013;18:657-65. [PubMed]
- Jin J, Joo KM, Lee SJ, et al. Synergistic therapeutic effects of cytokine-induced killer cells and temozolomide against glioblastoma. Oncol Rep 2011;25:33-9. [PubMed]
- Pelzer U, Schwaner I, Stieler J, et al. Best supportive care (BSC) versus oxaliplatin, folinic acid and 5-fluorouracil (OFF) plus BSC in patients for second-line advanced pancreatic cancer: a phase III-study from the German CONKO-study group. Eur J Cancer 2011;47:1676-81. [PubMed]
- Boeck S, Weigang-Köhler K, Fuchs M, et al. Second-line chemotherapy with pemetrexed after gemcitabine failure in patients with advanced pancreatic cancer: a multicenter phase II trial. Ann Oncol 2007;18:745-51. [PubMed]
- Ko AH, Tempero MA, Shan YS, et al. A multinational phase 2 study of nanoliposomal irinotecan sucrosofate (PEP02, MM-398) for patients with gemcitabine-refractory metastatic pancreatic cancer. Br J Cancer 2013;109:920-5. [PubMed]
- Lollini PL, Cavallo F, Nanni P, et al. Vaccines for tumour prevention. Nat Rev Cancer 2006;6:204-16. [PubMed]
- Günes C, Rudolph KL. The role of telomeres in stem cells and cancer. Cell 2013;152:390-3. [PubMed]
- Mocellin S, Pooley KA, Nitti D. Telomerase and the search for the end of cancer. Trends Mol Med 2013;19:125-33. [PubMed]
- Hiyama E, Kodama T, Shinbara K, et al. Telomerase activity is detected in pancreatic cancer but not in benign tumors. Cancer Res 1997;57:326-31. [PubMed]
- Takahara A, Koido S, Ito M, et al. Gemcitabine enhances Wilms' tumor gene WT1 expression and sensitizes human pancreatic cancer cells with WT1-specific T-cell-mediated antitumor immune response. Cancer Immunol Immunother 2011;60:1289-97. [PubMed]
- Huff V. Wilms' tumours: about tumour suppressor genes, an oncogene and a chameleon gene. Nat Rev Cancer 2011;11:111-21. [PubMed]
- Sugiyama H. WT1 (Wilms' tumor gene 1): biology and cancer immunotherapy. Jpn J Clin Oncol 2010;40:377-87. [PubMed]
- Elisseeva OA, Oka Y, Tsuboi A, et al. Humoral immune responses against Wilms tumor gene WT1 product in patients with hematopoietic malignancies. Blood 2002;99:3272-9. [PubMed]
- Oka Y, Elisseeva OA, Tsuboi A, et al. Human cytotoxic T-lymphocyte responses specific for peptides of the wild-type Wilms' tumor gene (WT1) product. Immunogenetics 2000;51:99-107. [PubMed]
- Oka Y, Tsuboi A, Taguchi T, et al. Induction of WT1 (Wilms' tumor gene)-specific cytotoxic T lymphocytes by WT1 peptide vaccine and the resultant cancer regression. Proc Natl Acad Sci U S A 2004;101:13885-90. [PubMed]
- Koido S, Homma S, Okamoto M, et al. Treatment with chemotherapy and dendritic cells pulsed with multiple Wilms' tumor 1 (WT1)-specific MHC class I/II-restricted epitopes for pancreatic cancer. Clin Cancer Res 2014;20:4228-39. [PubMed]
- Galili U. The alpha-gal epitope and the anti-Gal antibody in xenotransplantation and in cancer immunotherapy. Immunol Cell Biol 2005;83:674-86. [PubMed]
- Galili U, Chen ZC, DeGeest K. Expression of alpha-gal epitopes on ovarian carcinoma membranes to be used as a novel autologous tumor vaccine. Gynecol Oncol 2003;90:100-8. [PubMed]
- Joziasse DH, Oriol R. Xenotransplantation: the importance of the Galalpha1,3Gal epitope in hyperacute vascular rejection. Biochim Biophys Acta 1999;1455:403-18. [PubMed]
- Galili U, Mandrell RE, Hamadeh RM, et al. Interaction between human natural anti-alpha-galactosyl immunoglobulin G and bacteria of the human flora. Infect Immun 1988;56:1730-7. [PubMed]
- Xu L, Zhou X, Xu L, et al. Survivin rs9904341 (G>C) polymorphism contributes to cancer risk: an updated meta-analysis of 26 studies. Tumour Biol 2014;35:1661-9. [PubMed]
- Kami K, Doi R, Koizumi M, et al. Survivin expression is a prognostic marker in pancreatic cancer patients. Surgery 2004;136:443-8. [PubMed]
- Hirohashi Y, Torigoe T, Maeda A, et al. An HLA-A24-restricted cytotoxic T lymphocyte epitope of a tumor-associated protein, survivin. Clin Cancer Res 2002;8:1731-9. [PubMed]
- Kubuschok B, Neumann F, Breit R, et al. Naturally occurring T-cell response against mutated p21 ras oncoprotein in pancreatic cancer. Clin Cancer Res 2006;12:1365-72. [PubMed]
- Kotera Y, Fontenot JD, Pecher G, et al. Humoral immunity against a tandem repeat epitope of human mucin MUC-1 in sera from breast, pancreatic, and colon cancer patients. Cancer Res 1994;54:2856-60. [PubMed]
- Wada S, Tsunoda T, Baba T, et al. Rationale for antiangiogenic cancer therapy with vaccination using epitope peptides derived from human vascular endothelial growth factor receptor 2. Cancer Res 2005;65:4939-46. [PubMed]
- Itakura J, Ishiwata T, Shen B, et al. Concomitant over-expression of vascular endothelial growth factor and its receptors in pancreatic cancer. Int J Cancer 2000;85:27-34. [PubMed]
- von Marschall Z, Cramer T, Höcker M, et al. De novo expression of vascular endothelial growth factor in human pancreatic cancer: evidence for an autocrine mitogenic loop. Gastroenterology 2000;119:1358-72. [PubMed]
- Argani P, Iacobuzio-Donahue C, Ryu B, et al. Mesothelin is overexpressed in the vast majority of ductal adenocarcinomas of the pancreas: identification of a new pancreatic cancer marker by serial analysis of gene expression (SAGE). Clin Cancer Res 2001;7:3862-8. [PubMed]
- Galili U. Anti-Gal: an abundant human natural antibody of multiple pathogeneses and clinical benefits. Immunology 2013;140:1-11. [PubMed]
- Niethammer AG, Lubenau H, Mikus G, et al. Double-blind, placebo-controlled first in human study to investigate an oral vaccine aimed to elicit an immune reaction against the VEGF-Receptor 2 in patients with stage IV and locally advanced pancreatic cancer. BMC Cancer 2012;12:361. [PubMed]
- Jaffee EM, Hruban RH, Biedrzycki B, et al. Novel allogeneic granulocyte-macrophage colony-stimulating factor-secreting tumor vaccine for pancreatic cancer: a phase I trial of safety and immune activation. J Clin Oncol 2001;19:145-56. [PubMed]
- Le DT, Brockstedt DG, Nir-Paz R, et al. A live-attenuated Listeria vaccine (ANZ-100) and a live-attenuated Listeria vaccine expressing mesothelin (CRS-207) for advanced cancers: phase I studies of safety and immune induction. Clin Cancer Res 2012;18:858-68. [PubMed]
- Pardoll DM. The blockade of immune checkpoints in cancer immunotherapy. Nat Rev Cancer 2012;12:252-64. [PubMed]
- Hodi FS, Lee S, McDermott DF, et al. Ipilimumab plus sargramostim vs ipilimumab alone for treatment of metastatic melanoma: a randomized clinical trial. JAMA 2014;312:1744-53. [PubMed]
- Yang JC, Hughes M, Kammula U, et al. Ipilimumab (anti-CTLA4 antibody) causes regression of metastatic renal cell cancer associated with enteritis and hypophysitis. J Immunother 2007;30:825-30. [PubMed]
- Slovin SF, Higano CS, Hamid O, et al. Ipilimumab alone or in combination with radiotherapy in metastatic castration-resistant prostate cancer: results from an open-label, multicenter phase I/II study. Ann Oncol 2013;24:1813-21. [PubMed]
- Mocellin S, Nitti D. CTLA-4 blockade and the renaissance of cancer immunotherapy. Biochim Biophys Acta 2013;1836:187-96.
- Hurwitz AA, Yu TF, Leach DR, et al. CTLA-4 blockade synergizes with tumor-derived granulocyte-macrophage colony-stimulating factor for treatment of an experimental mammary carcinoma. Proc Natl Acad Sci U S A 1998;95:10067-71. [PubMed]
- van Elsas A, Hurwitz AA, Allison JP. Combination immunotherapy of B16 melanoma using anti-cytotoxic T lymphocyte-associated antigen 4 (CTLA-4) and granulocyte/macrophage colony-stimulating factor (GM-CSF)-producing vaccines induces rejection of subcutaneous and metastatic tumors accompanied by autoimmune depigmentation. J Exp Med 1999;190:355-66. [PubMed]
- Kameshima H, Tsuruma T, Torigoe T, et al. Immunogenic enhancement and clinical effect by type-I interferon of anti-apoptotic protein, survivin-derived peptide vaccine, in advanced colorectal cancer patients. Cancer Sci 2011;102:1181-7. [PubMed]
- Tsuruma T, Hata F, Torigoe T, et al. Phase I clinical study of anti-apoptosis protein, survivin-derived peptide vaccine therapy for patients with advanced or recurrent colorectal cancer. J Transl Med 2004;2:19. [PubMed]
- Kindler HL, Ioka T, Richel DJ, et al. Axitinib plus gemcitabine versus placebo plus gemcitabine in patients with advanced pancreatic adenocarcinoma: a double-blind randomised phase 3 study. Lancet Oncol 2011;12:256-62. [PubMed]
- Conroy T, Desseigne F, Ychou M, et al. FOLFIRINOX versus gemcitabine for metastatic pancreatic cancer. N Engl J Med 2011;364:1817-25. [PubMed]
- Von Hoff DD, Ervin T, Arena FP, et al. Increased survival in pancreatic cancer with nab-paclitaxel plus gemcitabine. N Engl J Med 2013;369:1691-703. [PubMed]
- Moore MJ, Goldstein D, Hamm J, et al. Erlotinib plus gemcitabine compared with gemcitabine alone in patients with advanced pancreatic cancer: a phase III trial of the National Cancer Institute of Canada Clinical Trials Group. J Clin Oncol 2007;25:1960-6. [PubMed]
- Drake CG. Combination immunotherapy approaches. Ann Oncol 2012;23:viii41-6. [PubMed]
- Zitvogel L, Apetoh L, Ghiringhelli F, et al. The anticancer immune response: indispensable for therapeutic success? J Clin Invest 2008;118:1991-2001. [PubMed]
- Formenti SC, Demaria S. Combining radiotherapy and cancer immunotherapy: a paradigm shift. J Natl Cancer Inst 2013;105:256-65. [PubMed]
- Wedén S, Klemp M, Gladhaug IP, et al. Long-term follow-up of patients with resected pancreatic cancer following vaccination against mutant K-ras. Int J Cancer 2011;128:1120-8. [PubMed]