HOXC10 up-regulation promotes gastric cancer cell proliferation and metastasis through MAPK pathway
Introduction
Although the incidence has been declining for several decades, gastric cancer was the leading cause of cancer-related deaths worldwide until the mid-1990s (1). In China, gastric cancer was the most common type of cancer and the second leading cause of cancer-related deaths after lung cancer until now (2). Therefore, there is an urgent need to identify novel prognostic and predictive biomarkers to improve the diagnosis and clinical management of patients with gastric cancer, which in turn may help to develop more effective treatment strategies.
In humans, 39 homeobox (HOX) genes are present, and organized into four different genomic clusters (HOX A–D) located on four chromosomes (7, 17, 12 and 2) (3). HOX genes are highly conserved at the genomic level and have been well-described as important players in regulating numerous processes including apoptosis, receptor signaling, differentiation, motility, angiogenesis, and metastasis (4,5). Aberrations in HOX gene expression have been reported in numerous malignancies, including ovarian cancer, esophageal squamous cell carcinoma, breast cancer, lung cancer, colorectal cancer, and hematological malignancies, and have been associated with significantly enhanced invasiveness, proliferation, and colony formation of tumor cells (6-12). In gastric cancer, previous data showed that approximately 20–25 HOX genes are expressed at least two-fold higher than in normal tissues (13), especially homeobox gene HOXB5 whose up-regulation induces invasion and migration through direct transcription and up-regulation of β-catenin in human gastric carcinoma (14). These results indicate that HOX genes can be considered as new targets for future tumor therapies.
HOXC10 is a member of the HOX gene family and significantly enhances proliferation, invasion, and metastasis of cancer cells, thus it might be useful as a marker for cancer diagnosis or progression. In cervical squamous cell carcinomas, elevated HOXC10 expression is associated with increased invasiveness as identified by using high-density oligonucleotide microarrays (15). In the Cancer Genome Atlas (TCGA) datasets, HOXC10 expression is significantly increased in human thyroid cancer tissues compared to normal human thyroid tissues. Furthermore, HOXC10 promotes migration and invasion of thyroid cancer cells, suggesting a role as a novel biomarker for human thyroid cancer prognosis (16), and promotes the metastasis of human lung adenocarcinoma and indicates poor survival outcome (17). However, the role of HOXC10 in breast cancer remains controversial (18-20). Remarkably, the latest research about the function of miR-136 in the gastric cancer cell line GC-9811 shows that HOXC10 can work as a direct target of miR-136, which inhibits gastric cancer-specific peritoneal metastasis. This indicates that HOXC10 functions as a metastasis promoter in gastric cancer peritoneal metastasis (21). However, there is no definite data to confirm the role of HOXC10 in the carcinogenesis and progression of gastric cancer.
In the present study, we discovered that HOXC10 expression was significantly increased in human gastric cancer tissues compared with normal tissues. In addition, HOXC10 could significantly promote the tumorigenicity of gastric cancer cells in vivo. Furthermore, HOXC10 knockdown or over-expression further confirmed that HOXC10 was required for proliferation, migration, and invasion of gastric cancer cells. With regard to its mechanism of action, our study showed that HOXC10 exerted its action on cancer through the mitogen-activated protein kinase (MAPK) pathway. Thus, HOXC10 might be considered as a potential target in the treatment of gastric cancer and as a marker in the prognosis of gastric cancer.
Materials and methods
Tissue samples and cell cultures
The biospecimens used in this study were provided by the Peking University Shenzhen Hospital. Gastric tissue from gastric cancer patients was divided into cancer tissue and adjacent normal tissue. Human gastric cancer cell lines AGS and SNU638 were purchased from American Type Culture Collection (ATCC) and were cultured in RPMI 1640 medium (Lonza, Walkersville, MD, USA) or Dulbecco’s modified Eagle’s medium (DMEM) (Lonza) supplemented with 10% (V/V) heat-inactivated fetal bovine serum (FBS) (Gibco-BRL) and 1% penicillin/streptomycin (Gibco-BRL). Cells were incubated at 37 °C in a 5% CO2 humidified atmosphere.
Animal experiments
A total of 20 eight-week-old male BALB/c nude mice weighing 18–20 g were purchased from the Department of Laboratory Animal Science, Peking University and, the protocols used in this study were approved by the Animal Care and Use Committee of Peking University (No. LA 2010-066). Plasmid pcDNA3-HOXC10 and control vector pcDNA3 were separately transfected into AGS cells to establish stable HOXC10-overexpressing cells (pcDNA3-HOXC10) and control cells (pcDNA3). Mice were anesthetized with isoflurane, the area on the midside of the abdomen was sterilized and the stomach was exposed via laparoscopy. A density of (5×106) cells per cell line (pcDNA3-HOXC10 or pcDNA3 control cells) were suspended in 50 μL of Hank’s balanced salt solution and placed into a sterile 31-gauge needle. The gauge tip was introduced 1.5 mm into the lesser curvature side of the gastric low body with an approximate 10° angle and the cell suspension was slowly injected (the procedure was performed under a binocular lens). Afterward, slight pressure was applied at 5 mm from the injection point using a gauze. The syringe needle was removed and the area around the injection was cleaned with 70% ethanol to avoid seeding of unlikely refluxed tumor cells into the abdominal cavity. After injection, the stomach was placed back into the abdominal cavity and the abdomen was closed with surgical drapes. Animals were sacrificed 5 weeks after surgery, and stomachs were examined. Tumors were measured at the indicated time points using calipers, and tumor volumes were calculated using the following formula: 1/2×length×width2.
Histology and immunohistochemical staining
Paraffin-embedded human gastric sections (4 μm thickness) were prepared via routine procedure (22). Immunohistochemical staining was performed using anti-HOXC10 polyclonal antibody (1:200, Santa Cruz Biotechnology, USA). After incubation with primary antibody at 4 °C overnight, slides were stained with horseradish peroxidase conjugated secondary antibody (DAKO, Denmark). Nonimmune normal IgG was used to replace the primary antibody as a negative control. Slides were viewed under a Leica TCS SP8 confocal microscope.
Protein arrays and western blotting analysis
Detection of 46 phosphoproteins was performed on Proteome Profiler Human Phospho-Kinase Array Kit membranes (R&D Systems, Minneapolis, MN, USA) according to manufacturer’s instructions. Whole-cell lysates were obtained using RIPA buffer containing protease inhibitor and phosphatase inhibitor (GenDEPOT). Protein concentration was measured using a BCA Protein Assay Kit (Pierce). Western blotting was performed using 10 μg/lane of total protein and specific proteins were detected using the primary antibodies listed below, glyceraldehyde-3-phosphate dehydrogenase (GAPDH) was used as a loading control. Horseradish peroxidase-conjugated secondary antibodies were purchased from Jackson ImmunoResearch (West Grove, PA, USA). Signals were detected using West Pico or West Femto reagents (Pierce, Waltham, MA, USA) and a FujiFilm LAS-3000 imager (Tokyo, Japan). Band quantification was performed using Image-J software from raw image files according to manufacturer’s instructions. The following antibodies were used: anti-JNK, anti-p-JNK, anti-P38, anti-p-P38, anti-ERK, anti-p-ERK, anti-c-Myc, anti-β-catenin, and anti-P53 from Cell Signaling Technology, anti-GAPDH, anti-RAC1, and anti-c-Jun from Santa Cruz Biotechnology, and anti-HOXC10 from Proteintech for western blotting.
Transfection assay
To generate the plasmid containing the HOXC10 gene, a 1.29 kb polymerase chain reaction (PCR) fragment containing full-length HOXC10 was amplified from cDNA (gift from Professor Han Jiahuai’s Lab) using the following primers; forward 5’-ATCGAATTCATGACATGCCCTCGCAATGT-3’ and reverse 5’-ATCCTCGAGTCAGGTGAAATTAAAATTGG-3’ (with the additionally introduced restriction sites of EcoRI and XhoI underlined). A pcDNA3-HOXC10 plasmid was constructed by cloning the PCR fragment into the pcDNA3 at EcoRI and XhoI sites. Transfection was performed using LipofectamineTM 3000 (Invitrogen) in AGS cell of 80% concentration growth according to the manufacturer’s instructions. At 48 h after transfection, 5 μg/mL blasticidin (Sigma) was added, and live cells were selected as stable transfected cells. To knockdown HOXC10 expression, siRNA against HOXC10 (santa cruz, sc-44810) and negative control siRNA (SN-1003) 60 nmol/L were transiently transfected using RNAiMAX (Invitrogen) according to the manufacturer’s protocol. HOXC10 up- and down-regulations were assessed via reverse transcription PCR (RT-PCR) and western blotting.
Cell proliferation assay
A total of 3,000 cells per well were plated in 96-well plates in triplicate and 3,(4,5-dimethylthizol-2-yl)-2,5-diphenyltetrazolium bromide (MTT) assay was performed following manufacturer’s instructions. In short, 10 μL of 10 mg/mL MTT solution was added to each well and incubated for 2 h at 37 °C. Optical density (OD) was measured using a microplate reader at a 490-nm wavelength.
Migration and invasion assays
Cell migration was measured using Culture-Inserts (Ibidi, Regensburg, Germany). The Culture-Inserts were transferred into 6-well culture plates and cells were seeded at a density of 5×104 cell/100 μL in each well of Culture-Inserts.
After 24 h incubation, the Culture-Inserts were removed, and cell-free gaps were created. Images of the closed gaps were captured at the indicated incubation time. Cell invasion assays were conducted using transwell filters chambers that were coated with 1% gelatin in culture media overnight and dried at room temperature.
Cells were seeded at 2×105 cells in 150 μL medium without bovine serum albumin (BSA) on the upper chamber. Then, 500 μL medium with 5% BSA was loaded into the lower chamber. After 24 h of incubation, cells that had invaded the bottom surface of the transwell were fixed with 4% paraformaldehyde (PFA), stained with Diff Quik solution (Sysmex), and counted in five selected fields. Both assays were performed as perviously reported (14,23).
Statistical analysis
All statistical analyses were performed using IBM SPSS Statistics (Version 20.0; IBM Corp., New York, USA). Statistical significance was assessed via Student’s t-test or Mann-Whitney U test, as appropriate. The significant difference between multiple groups was determined using 2-way analysis of variance (ANOVA). P-values below 0.05 were considered statistically significant.
Results
HOXC10 is increased in gastric cancer tissues
To explore the role of HOXC10 in gastric cancer, we examined HOXC10 expression in 10 pairs of gastric cancer tissues and their adjacent non-tumor tissues using RT-PCR. HOXC10 mRNA was detected in all cancer tissues and in four (40%) of the 10 noncancerous tissues. In six (60%) of the 10 samples, HOXC10 mRNA expression was significantly higher in the cancerous tissue than in the noncancerous tissue and the difference was statistically significant (P<0.05,Figure 1A).
To further confirm this result, HOXC10 expression was analyzed via immunohistochemistry in primary gastric carcinoma specimens, cancer-adjacent normal gastric tissues, and gastric adenocarcinoma lymph node metastatic tissues. HOXC10 was mainly localized into the nucleus of the gastric lesions, with different staining intensities observed in different stages of gastric cancer (Figure 1B). Higher HOXC10 levels were expressed in gastric cancer compared to normal gastric mucosa.
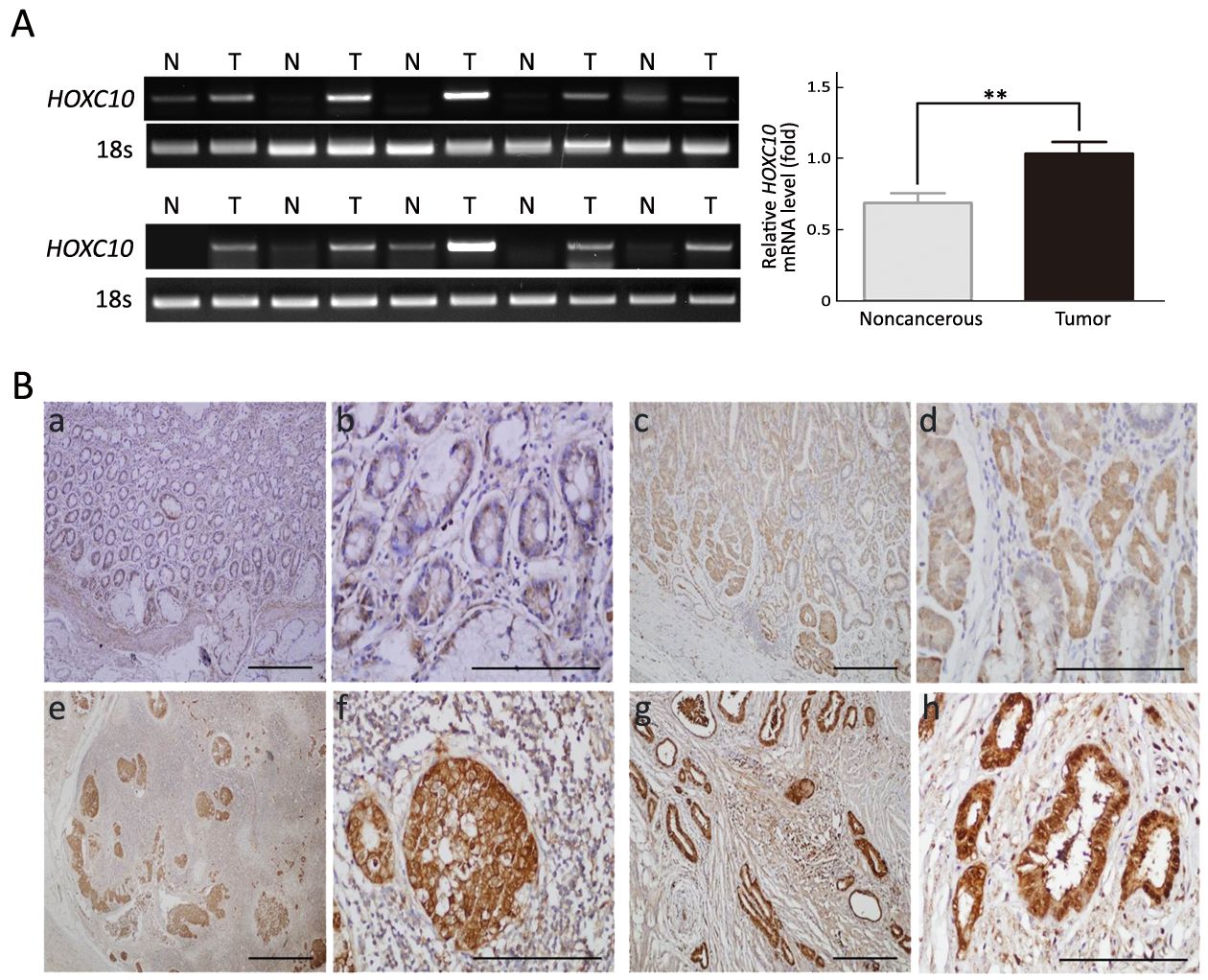
HOXC10 promotes primary gastric tumor growth and cell proliferation
AGS cells transfected with the plasmid containing HOXC10 (pcDNA3-HOXC10) showed a significant HOXC10 mRNA increase (10-fold) compared to control cells transfected with empty vector (pcDNA3) (P<0.001,Figure 2A). Western blotting showed the same protein expression level tendency (Figure 2C). This result indicated a successful transfection. In a separated experiment, HOXC10 mRNA expression in SNU638 cells was inhibited by specific siRNA against HOXC10. HOXC10 mRNA expression was significantly down-regulated in the siRNA-treated cells, compared to parental and control cells, at 48 h after transfection (P<0.001,Figure 2B). Protein levels were also assessed via western blotting (Figure 2D). Furthermore, we evaluated whether HOXC10 could promote primary gastric tumor growth in vivo. BALB/c nude mice underwent surgery according to the described methods (Figure 3A). Our results show that HOXC10 substantially increased primary tumor growth, as compared to control tumors (Figure 3B). Primary tumor weight was approximately 53% higher in mice overexpressing HOXC10, as compared to control mice (P<0.05,Figure 3C).
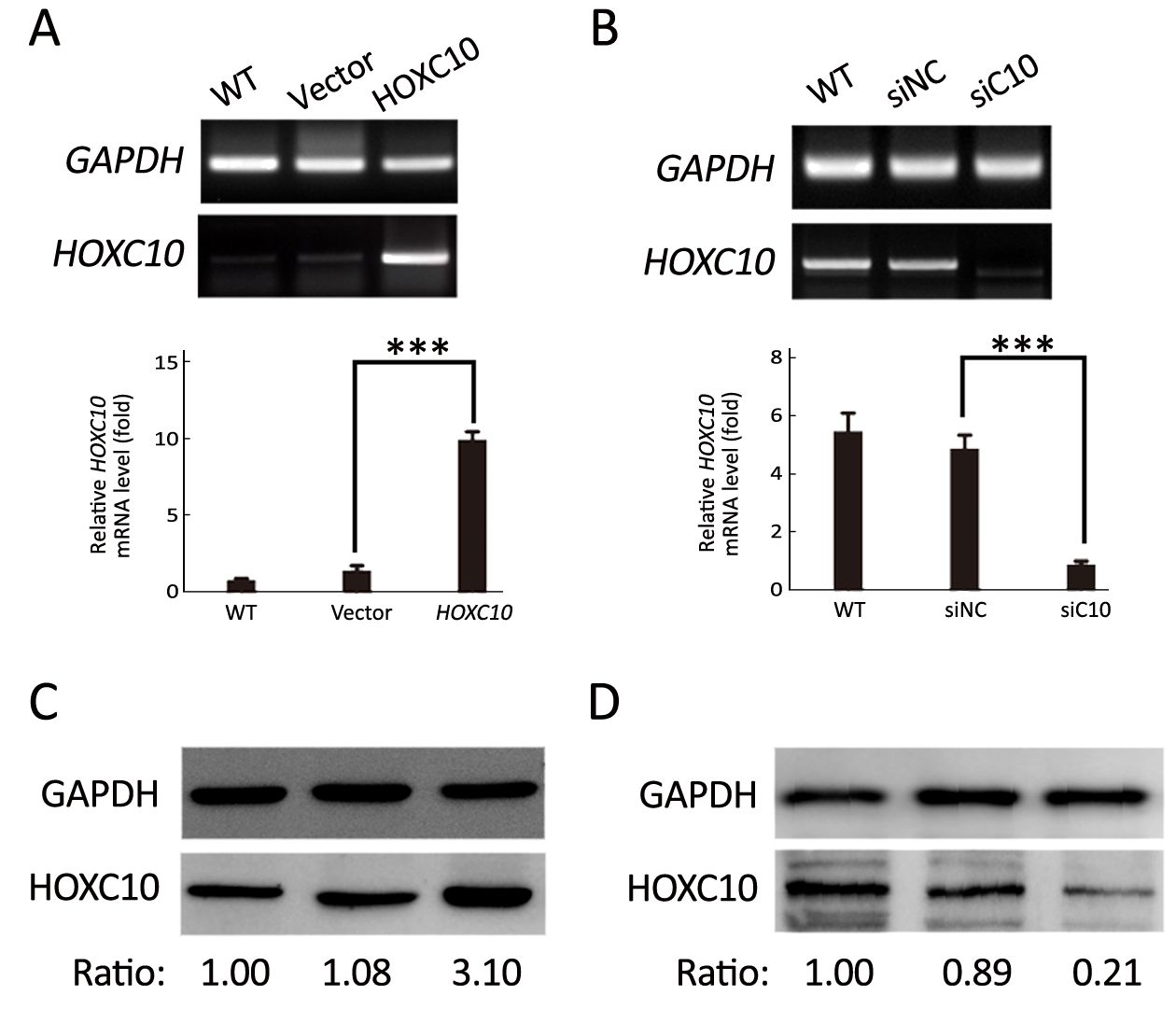
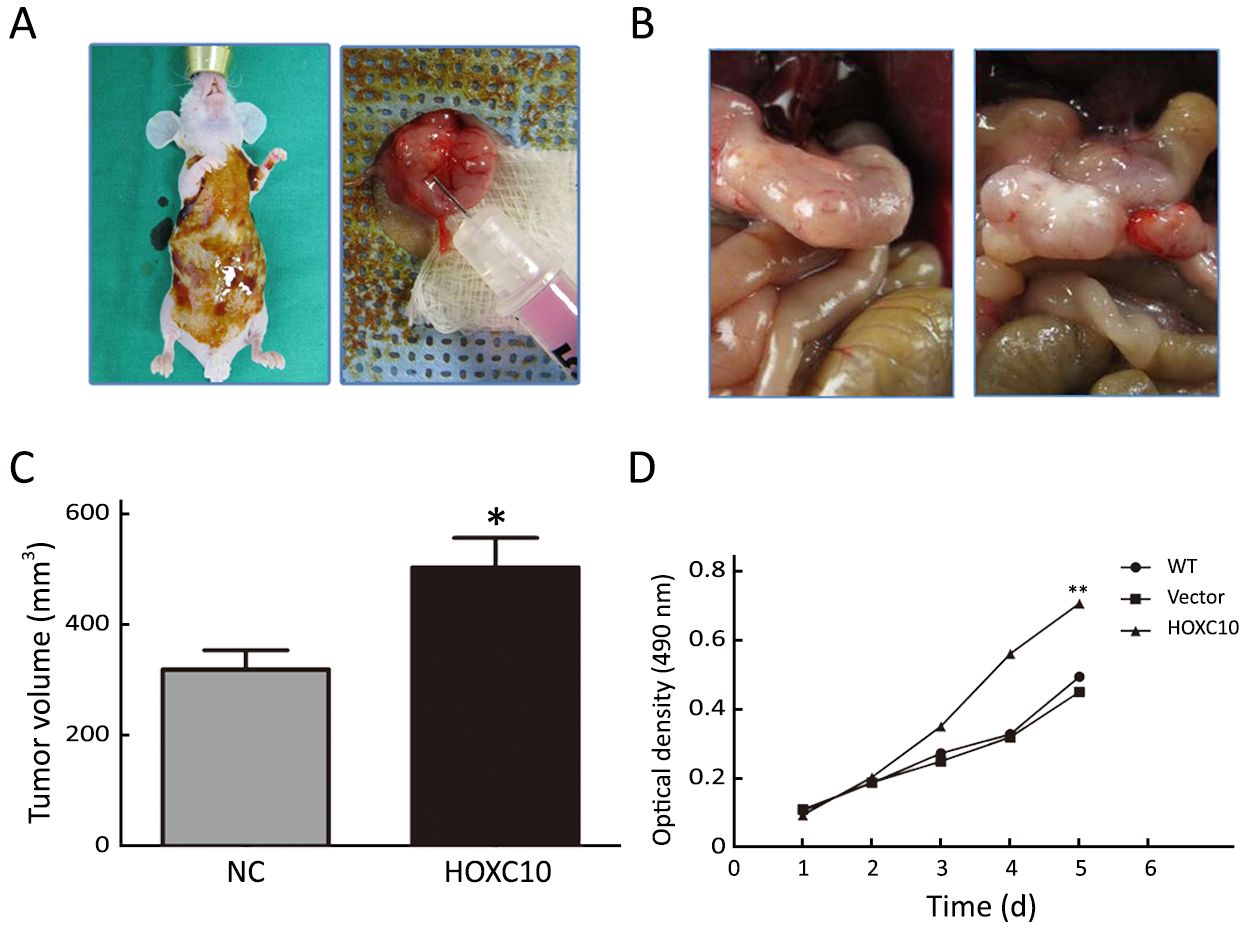
Moreover, cell viability results demonstrated that AGS cells transfected with pCDNA3-HOXC10 showed a significant increase in cell viability compared to the control (P<0.01,Figure 3D). In contrast, SNU638 cells transfected with siHOXC10 exhibited decreased cell viability; however, this effect was not significant. A likely reason is that the effect of siRNA cannot be maintained.
HOXC10 induces gastric cancer cell line invasion and migration
HOXC10 ectopic expression in AGS cells markedly enhanced cellular migration and invasion compared to untreated or empty vector transfected cells (P<0.001,Figure 4A, C). In contrast to untreated or scrambled siRNA control transfected SNU638 cells, HOXC10 knockdown significantly decreased the migration and invasion of SNU638 cells (P<0.0001,Figure 4B, D). These results demonstrate that HOXC10 promoted migration and invasion of gastric cancer cells.
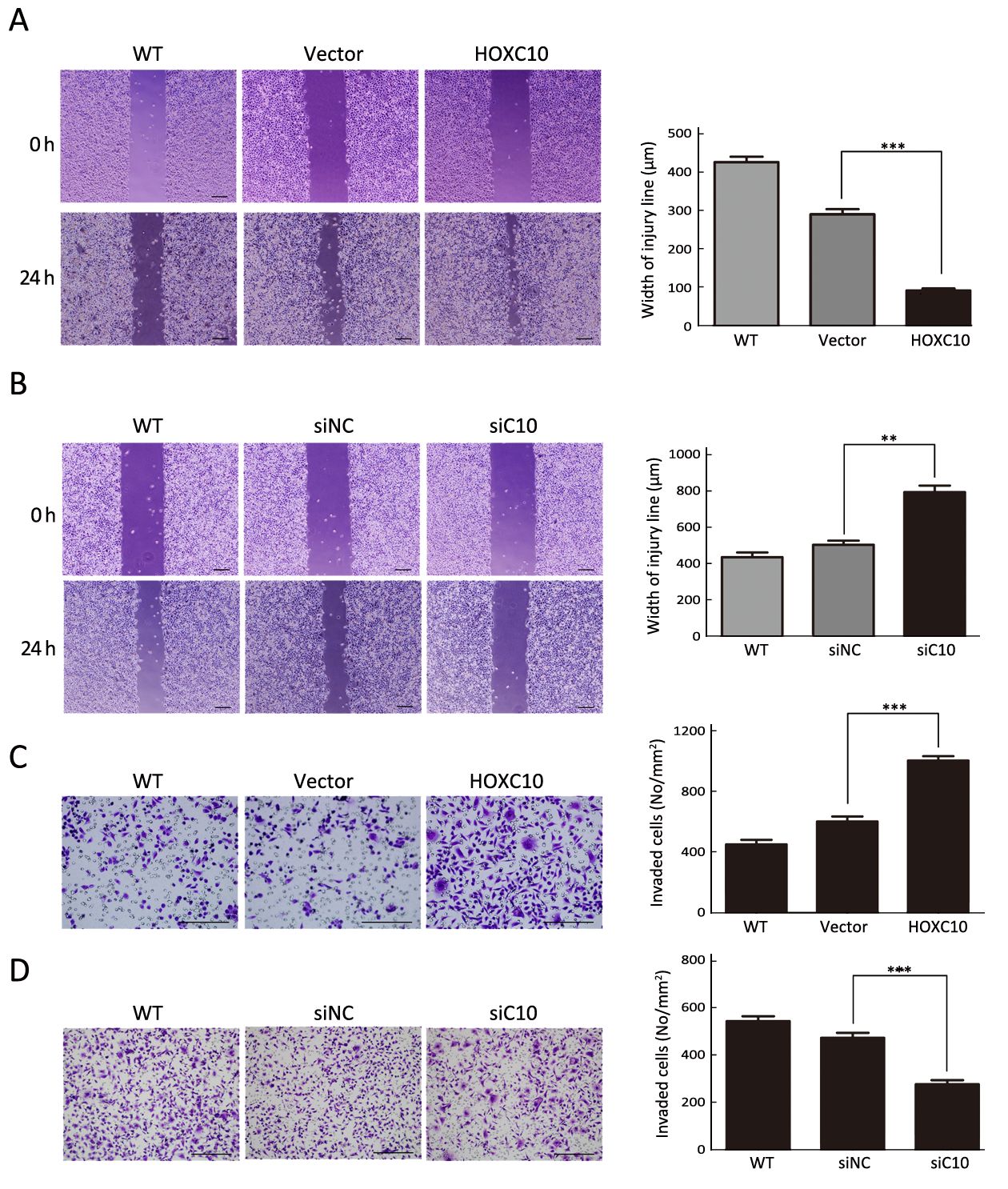
HOXC10 is associated with transcriptional activity of the MAPK pathway
Currently, the role of HOXC10-induced signaling pathways and HOXC10-associated kinases is not well understood in gastric cancer. Thus, the phosphorkinase array assay was performed. In Figure 5A, the phosphorylation of extracellular signal-regulated kinase (ERK)1/2, P38a, c-Jun N-terminal kinase (JNK)1/2/3, protein kinase B (AKT), and staphylococcal leucocidin (LUK) were increased in 100 ng protein lysis of AGS cells transfected with HOXC10 after 24 h of incubation, while the expression of β-catenin barely changed.
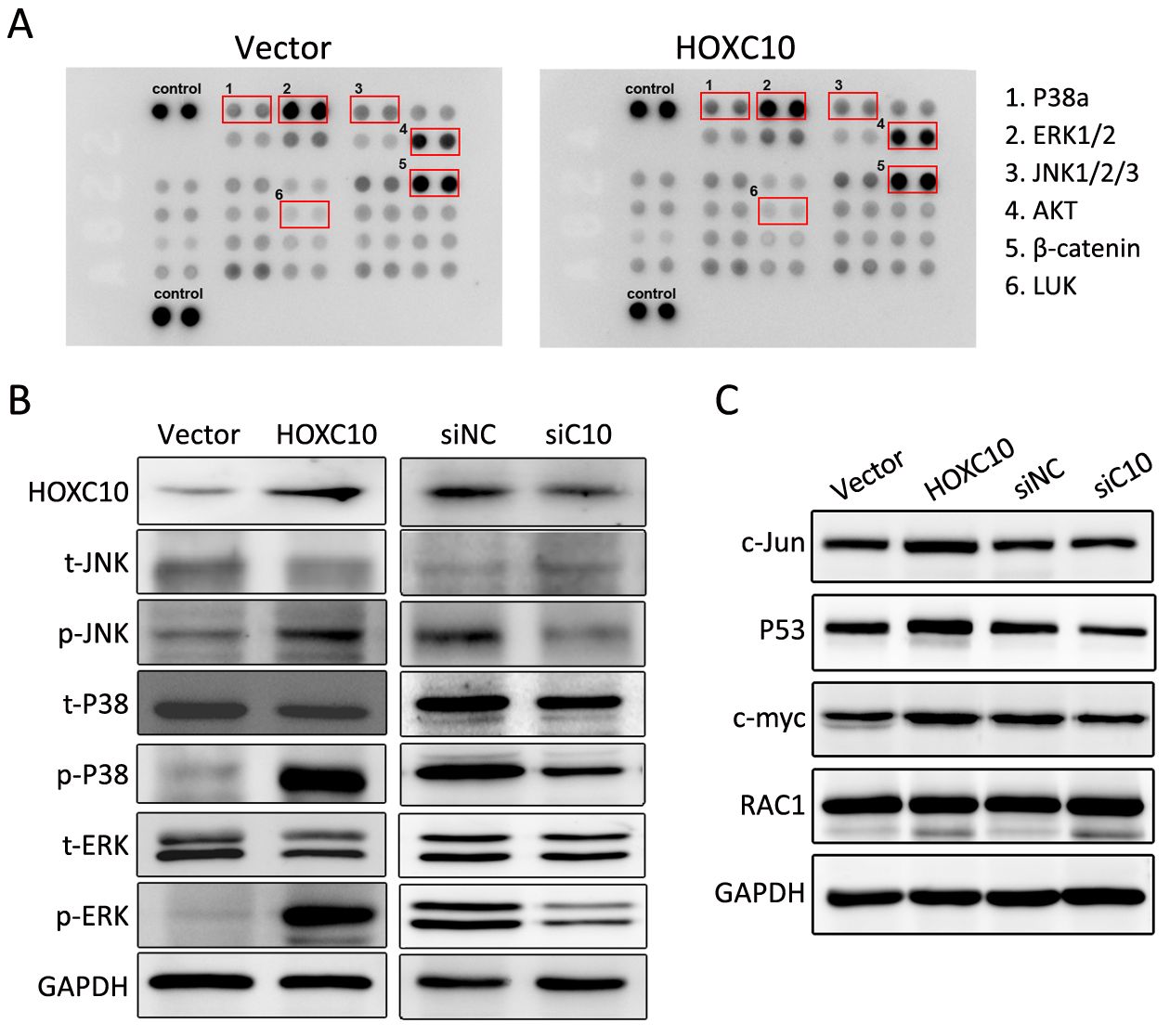
We further evaluated the phosphorylation of P38, JNK, and ERK1/2 in response to HOXC10 silencing or over-expression. Western blotting showed that HOXC10 significantly increased the phosphorylation of JNK or ERK1/2 and P38 (Figures 5B). As important downstream molecules of MAPK pathway, MYC proto-oncogene (c-myc), Jun proto-oncogene, AP-1 transcription factor subunit (c-Jun) and P53 are involved in cancer proliferation and metastasis. As expected, HOXC10 ectopic expression in AGS cells markedly increased c-myc, c-Jun and P53 protein expressions. At the same time, c-myc, c-Jun, and P53 protein levels were remarkably decreased in SNU638 cells after transfection with HOXC10 siRNA compared to scrambled siRNA control or untreated cells (Figures 5C). Since HOXC10 showed a remarkable effect of MAPK signaling, we also evaluated RAC1 expression, which is involved in the non-classical MAPK pathway and plays a role in metastasis. However, we did not find any significant change.
Discussion
HOX gene products are transcription factors responsible for regulating phenotype cell identity, differentiation, and controlling primary cellular processes (24). In addition to their function in embryonic development and tissue remodeling, the HOX gene network has been well-described as involved in hematopoiesis and leukemogenesis (25). Recently, inappropriate HOX gene expression has been associated with different neoplasias occurring in kidney, colon, lung, skin, bladder, liver, breast, and prostate. HOXC10, as a member of the HOX gene family, has recently received increased attention due to its important role in tumor development.
In this study, we show that HOXC10 is abnormally up-regulated in gastric cancer especially in tissues from gastric cancer patients with lymph node or distal metastasis, suggesting that its expression increased throughout tumor progression. Although more samples are required to verify the connection between the expression of HOXC10 and pathological and stage types of gastric cancer, our results demonstrated the role of HOXC10 for gastric cancer development. Similar results have been reported that elevated HOXC10 expression is associated with increased invasiveness and poor survival outcome in human cervical cancer and thyroid cancer (15,16). HOXC10 is also up-regulated following chemotherapy or ionizing radiation in estrogen receptor (ER) negative breast cancer. Then, as a part of the Cdk-activating kinase complex, HOXC10 participates in the late stages of DNA repair that involves restart of transcription for recovery and survival of cancer cells in response to chemotherapy (20). Thus, our study further highlighted the potential role of HOXC10 as a novel biomarker for human gastric cancer prognosis.
In order to explore how HOXC10 affects the biological functions of gastric cancer cells, we first conducted animal experiments, which showed that high HOXC10 expression could significantly improve the formation of gastric tumors. At a cellular level, high expression of HOXC10 enhanced gastric cancer cell proliferation while low expression decreased their proliferation. As early as 2003, the literature demonstrated that HOXC10 is associated with several transcription factors namely E2F, MyoD, Myc and c-Jun, known to be substrates for the ubiquitin proteasome pathway involved in proliferation control (26). Recent research showed that up-regulated HOXC10 expression promotes proliferation, invasion, and migration of osteosarcoma cell lines and inhibits their apoptosis (27). Therefore, we have come to the conclusion that HOXC10 also promotes the proliferation of gastric cancer cells.
In gastric cancer, metastasis is a crucial factor affecting tumor development and prognosis. We further studied the effect of HOXC10 on gastric cancer cell metastasis through invasion and migration assays, discovering that HOXC10 promoted gastric cancer cell metastasis. In our study, HOXC10 has been shown to be involved in tumor progression, including proliferation, migration, and invasion.
Since we discovered that HOXC10 occupies such an important function in gastric cancer cell proliferation and metastasis, a study of its regulatory mechanism was necessary. We first performed a protein array to screen the related pathway. As a result, change in HOXC10 expression affected several signaling pathways. The MAPK signaling pathway is well known for its role in controlling cellular processes such as proliferation, differentiation, and apoptosis (28). The p38 kinase, ERK, and the stress-activated protein kinase (SAPK)/JNK are the three most thoroughly explored MAPK pathways (29-31). Therefore, we focused on MAPK signaling. We evaluated MAPK signaling via western blotting, discovering that HOXC10 increase resulted in the phosphorylation of JNK/ERK/P38. As a consequence of this result, we analyzed the downstream targets that regulate cell proliferation and metastasis such as c-myc, c-jun and p53, and found that these genes were also up-regulated by HOXC10. We also evaluated whether HOXC10 affected the small GTPase modulating cell metastasis; however, the results showed that total RAC1 and active RAC1 were not different between HOXC10 overexpressing cells and control cells.
Conclusions
Overall, our study indicated a key role of HOXC10 in the proliferation and metastasis of gastric cancer cells. Moreover, HOXC10 regulated these biological progresses through MAPK signaling, thus providing useful information for targeted therapy against gastric cancer. Therefore, this more comprehensive understanding of the role of HOXC10 in gastric cancer might contribute to the development of new treatment methods.
Acknowledgements
This work was supported by the grants from ‘San Ming’ Project of Shenzhen city, China; and Municipal Health planning Commission Fund of Shenzhen city, China (No. 201601004 and No. 201704260051).
Footnote
Conflicts of Interest: The authors have no conflicts of interest to declare.
References
- Siegel RL, Miller KD, Jemal A. Cancer Statistics, 2017. CA Cancer J Clin 2017;67:7–30. DOI:10.3322/caac.21387
- Chen W, Zheng R, Zuo T, et al. National cancer incidence and mortality in China, 2012. Chin J Cancer Res 2016;28:1–11. DOI:10.3978/j.issn.1000-9604.2016.02.08
- Gehring WJ, Hiromi Y. Homeotic genes and the homeobox. Annu Rev Genet 1986;20:147–73. DOI:10.1146/annurev.ge.20.120186.001051
- Abate-Shen C. Deregulated homeobox gene expression in cancer: cause or consequence?. Nat Rev Cancer 2002;2:777–85. DOI:10.1038/nrc907
- Shah N, Sukumar S. The Hox genes and their roles in oncogenesis. Nat Rev Cancer 2010;10:361–71. DOI:10.1038/nrc2826
- Kar SP, Tyrer JP, Li Q, et al. Network-based integration of GWAS and gene expression identifies a HOX-centric network associated with serous ovarian cancer risk. Cancer Epidemiol Biomarkers Prev 2015;24:1574–84. DOI:10.1158/1055-9965.EPI-14-1270
- Tang W, Jiang Y, Mu X, et al. MiR-135a functions as a tumor suppressor in epithelial ovarian cancer and regulates HOXA10 expression. Cell Signal 2014;26:1420–6. DOI:10.1016/j.cellsig.2014.03.002
- Lin C, Wang Y, Wang Y, et al. Transcriptional and posttranscriptional regulation of HOXA13 by lncRNA HOTTIP facilitates tumorigenesis and metastasis in esophageal squamous carcinoma cells. Oncogene 2017;36:5392–406. DOI:10.1038/onc.2017.133
- Teo WW, Merino VF, Cho S, et al. HOXA5 determines cell fate transition and impedes tumor initiation and progression in breast cancer through regulation of E-cadherin and CD24. Oncogene 2016;35:5539–51. DOI:10.1038/onc.2016.95
- Li M, Li X, Zhuang Y, et al. Induction of HOXA9 expression in three-dimensional organotypic culture of the Claudin-low breast cancer cells. Oncotarget 2016;7:51503–14. DOI:10.18632/oncotarget.10491
- Mansour MA, Senga T. HOXD8 exerts a tumor-suppressing role in colorectal cancer as an apoptotic inducer. Int J Biochem Cell Biol 2017;88:1–13. DOI:10.1016/j.biocel.2017.04.011
- Abe M, Hamada J, Takahashi O, et al. Disordered expression of HOX genes in human non-small cell lung cancer. Oncol Rep 2006;15:797–802.
- Yahagi N, Kosaki R, Ito T, et al. Position-specific expression of Hox genes along the gastrointestinal tract. Congenit Anom (Kyoto) 2004;44:18–26.
- Hong CS, Jeong O, Piao Z, et al. HOXB5 induces invasion and migration through direct transcriptional up-regulation of beta-catenin in human gastric carcinoma. Biochem J 2015;472:393–403. DOI:10.1042/BJ20150213
- Zhai Y, Kuick R, Nan B, et al. Gene expression analysis of preinvasive and invasive cervical squamous cell carcinomas identifies HOXC10 as a key mediator of invasion. Cancer Res 2007;67:10163–72. DOI:10.1158/0008-5472.CAN-07-2056
- Feng X, Li T, Liu Z, et al. HOXC10 up-regulation contributes to human thyroid cancer and indicates poor survival outcome. Mol Biosyst 2015;11:2946–54. DOI:10.1039/c5mb00253b
- Tang XL, Ding BX, Hua Y, et al. HOXC10 promotes the metastasis of human lung adenocarcinoma and indicates poor survival outcome. Front Physiol 2017;8:557. DOI:10.3389/fphys.2017.00557
- Ansari KI, Hussain I, Kasiri S, et al. HOXC10 is overexpressed in breast cancer and transcriptionally regulated by estrogen via involvement of histone methylases MLL3 and MLL4. J Mol Endocrinol 2012;48:61–75. DOI:10.1530/JME-11-0078
- Pathiraja TN, Nayak SR, Xi Y, et al. Epigenetic reprogramming of HOXC10 in endocrine-resistant breast cancer. Sci Transl Med 2014;6:229ra41. DOI:10.1126/scitranslmed.3008326
- Sadik H, Korangath P, Nguyen NK, et al. HOXC10 expression supports the development of chemotherapy resistance by fine tuning DNA repair in breast cancer cells. Cancer Res 2016;76:4443–56. DOI:10.1158/0008-5472.CAN-16-0774
- Zheng J, Ge P, Liu X, et al. MiR-136 inhibits gastric cancer-specific peritoneal metastasis by targeting HOXC10. Tumour Biol 2017;39:1010428317706207. DOI:10.1177/1010428317706207
- Wang J, Wang T, Xu J, et al. Prognostic significance of X-ray cross-complementing gene 1 expression in gastric cancer. Chin J Cancer Res 2016;28:355–61. DOI:10.21147/j.issn.1000-9604.2016.03.10
- Piao Z, Hong CS, Jung MR, et al. Thymosin β4 induces invasion and migration of human colorectal cancer cells through the ILK/AKT/β-catenin signaling pathway. Biochem Biophys Res Commun 2014;452:858–64. DOI:10.1016/j.bbrc.2014.09.012
- Anbazhagan R, Raman V. Homeobox genes: molecular link between congenital anomalies and cancer. Eur J Cancer 1997;33:635–7.
- Argiropoulos B, Humphries RK. Hox genes in hematopoiesis and leukemogenesis. Oncogene 2007;26:6766–76. DOI:10.1038/sj.onc.1210760
- Gabellini D, Colaluca IN, Vodermaier HC, et al. Early mitotic degradation of the homeoprotein HOXC10 is potentially linked to cell cycle progression. EMBO J 2003;22:3715–24. DOI:10.1093/emboj/cdg340
- Xiong W, Zhou Q, Liu G, et al. Homeodomain-containing gene 10 inhibits cell apoptosis and promotes cell invasion and migration in osteosarcoma cell lines. Tumour Biol 2017;39:1010428317697566. DOI:10.1177/1010428317697566
- Kim EK, Choi EJ. Compromised MAPK signaling in human diseases: an update. Arch Toxicol 2015;89:867–82. DOI:10.1007/s00204-015-1472-2
- Peluso I, Yarla NS, Ambra R, et al. MAPK signalling pathway in cancers: Olive products as cancer preventive and therapeutic agents. Semin Cancer Biol 2017 Sep 11. DOI:10.1016/j.semcancer.2017.09.002
- Ki MR, Lee HR, Goo MJ, et al. Differential regulation of ERK1/2 and p38 MAP kinases in VacA-induced apoptosis of gastric epithelial cells. Am J Physiol Gastrointest Liver Physiol 2008;294:G635–47. DOI:10.1152/ajpgi.00281.2007
- Katsoulidis E, Li Y, Mears H, et al. The p38 mitogen-activated protein kinase pathway in interferon signal transduction. J Interferon Cytokine Res 2005;25:749–56. DOI:10.1089/jir.2005.25.749